Hyperoxia Disrupts Lung Lymphatic Homeostasis in Neonatal Mice
- PMID: 36978868
- PMCID: PMC10045755
- DOI: 10.3390/antiox12030620
Hyperoxia Disrupts Lung Lymphatic Homeostasis in Neonatal Mice
Abstract
Inflammation causes bronchopulmonary dysplasia (BPD), a common lung disease of preterm infants. One reason this disease lacks specific therapies is the paucity of information on the mechanisms regulating inflammation in developing lungs. We address this gap by characterizing the lymphatic phenotype in an experimental BPD model because lymphatics are major regulators of immune homeostasis. We hypothesized that hyperoxia (HO), a major risk factor for experimental and human BPD, disrupts lymphatic endothelial homeostasis using neonatal mice and human dermal lymphatic endothelial cells (HDLECs). Exposure to 70% O2 for 24-72 h decreased the expression of prospero homeobox 1 (Prox1) and vascular endothelial growth factor c (Vegf-c) and increased the expression of heme oxygenase 1 and NAD(P)H dehydrogenase [quinone]1 in HDLECs, and reduced their tubule formation ability. Next, we determined Prox1 and Vegf-c mRNA levels on postnatal days (P) 7 and 14 in neonatal murine lungs. The mRNA levels of these genes increased from P7 to P14, and 70% O2 exposure for 14 d (HO) attenuated this physiological increase in pro-lymphatic factors. Further, HO exposure decreased VEGFR3+ and podoplanin+ lymphatic vessel density and lymphatic function in neonatal murine lungs. Collectively, our results validate the hypothesis that HO disrupts lymphatic endothelial homeostasis.
Keywords: bronchopulmonary dysplasia; human dermal lymphatic endothelial cells; hyperoxia; lymphangiogenesis; lymphatic function.
Conflict of interest statement
The authors declare no conflict of interest.
Figures
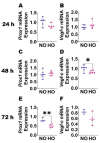
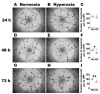
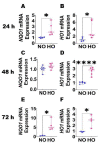
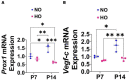
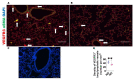
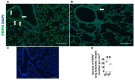
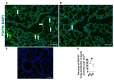
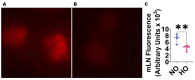
Similar articles
-
Upregulation of Vascular Endothelial Growth Factor in Amniotic Fluid Stem Cells Enhances Their Potential to Attenuate Lung Injury in a Preterm Rabbit Model of Bronchopulmonary Dysplasia.Neonatology. 2018;113(3):275-285. doi: 10.1159/000481794. Epub 2018 Feb 1. Neonatology. 2018. PMID: 29393249
-
[Effect of intra-amniotic endotoxin priming plus hyperoxic exposure on the expression of vascular endothelial growth factor and its receptors in lungs of preterm newborn rats].Zhonghua Er Ke Za Zhi. 2007 Jul;45(7):533-8. Zhonghua Er Ke Za Zhi. 2007. PMID: 17953812 Chinese.
-
Adiponectin ameliorates hyperoxia-induced lung endothelial dysfunction and promotes angiogenesis in neonatal mice.Pediatr Res. 2022 Feb;91(3):545-555. doi: 10.1038/s41390-021-01442-5. Epub 2021 Mar 25. Pediatr Res. 2022. PMID: 33767374
-
Endothelial Adenosine Monophosphate-Activated Protein Kinase-Alpha1 Deficiency Potentiates Hyperoxia-Induced Experimental Bronchopulmonary Dysplasia and Pulmonary Hypertension.Antioxidants (Basel). 2021 Nov 29;10(12):1913. doi: 10.3390/antiox10121913. Antioxidants (Basel). 2021. PMID: 34943016 Free PMC article.
-
Endometrial lymphangiogensis.Placenta. 2008 Mar;29 Suppl A:S48-54. doi: 10.1016/j.placenta.2007.09.009. Epub 2007 Nov 5. Placenta. 2008. PMID: 17981324 Review.
Cited by
-
Oxidative Stress in Fetuses and Newborns.Antioxidants (Basel). 2024 Sep 25;13(10):1157. doi: 10.3390/antiox13101157. Antioxidants (Basel). 2024. PMID: 39456411 Free PMC article.
-
Mycoplasma pneumoniae pneumonia-associated thromboembolism with plastic bronchitis: a series of five case reports and literature review.Ital J Pediatr. 2024 Jun 18;50(1):117. doi: 10.1186/s13052-024-01690-1. Ital J Pediatr. 2024. PMID: 38886770 Free PMC article. Review.
References
Grants and funding
LinkOut - more resources
Full Text Sources
Research Materials
Miscellaneous