Characterisation of the immune repertoire of a humanised transgenic mouse through immunophenotyping and high-throughput sequencing
- PMID: 36971345
- PMCID: PMC10115447
- DOI: 10.7554/eLife.81629
Characterisation of the immune repertoire of a humanised transgenic mouse through immunophenotyping and high-throughput sequencing
Abstract
Immunoglobulin loci-transgenic animals are widely used in antibody discovery and increasingly in vaccine response modelling. In this study, we phenotypically characterised B-cell populations from the Intelliselect Transgenic mouse (Kymouse) demonstrating full B-cell development competence. Comparison of the naïve B-cell receptor (BCR) repertoires of Kymice BCRs, naïve human, and murine BCR repertoires revealed key differences in germline gene usage and junctional diversification. These differences result in Kymice having CDRH3 length and diversity intermediate between mice and humans. To compare the structural space explored by CDRH3s in each species' repertoire, we used computational structure prediction to show that Kymouse naïve BCR repertoires are more human-like than mouse-like in their predicted distribution of CDRH3 shape. Our combined sequence and structural analysis indicates that the naïve Kymouse BCR repertoire is diverse with key similarities to human repertoires, while immunophenotyping confirms that selected naïve B cells are able to go through complete development.
Keywords: B cell; antibody; human; immunology; inflammation; mouse; transgenic mouse.
© 2023, Richardson, Binter et al.
Conflict of interest statement
ER Eve Richardson receives funding from Kymab Ltd, ŠB Špela Binter is an employee of Kymab Ltd, MK Miha Kosmac was an employee of Kymab within the last three years, MG, Vv, AK, JT, DK, CD No competing interests declared, JG Employee of Alchemab Therapeutics Ltd, PK Paul Kellam is an employee of Kymab Ltd, SW Simon Watson is an employee of Kymab Ltd
Figures
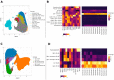
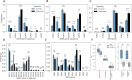
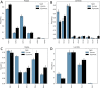
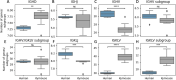
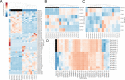
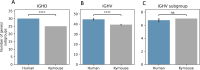
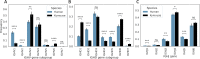
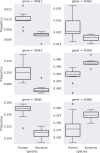
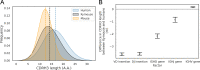
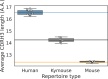
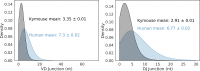
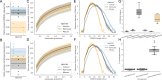
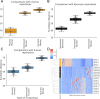
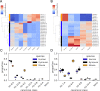
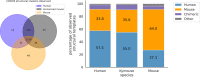
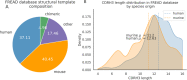
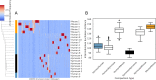
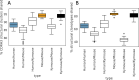
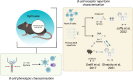
Update of
- doi: 10.1101/2022.06.27.497709
Similar articles
-
Structural diversity of B-cell receptor repertoires along the B-cell differentiation axis in humans and mice.PLoS Comput Biol. 2020 Feb 18;16(2):e1007636. doi: 10.1371/journal.pcbi.1007636. eCollection 2020 Feb. PLoS Comput Biol. 2020. PMID: 32069281 Free PMC article.
-
How repertoire data are changing antibody science.J Biol Chem. 2020 Jul 17;295(29):9823-9837. doi: 10.1074/jbc.REV120.010181. Epub 2020 May 14. J Biol Chem. 2020. PMID: 32409582 Free PMC article. Review.
-
Computational Analysis of B-Cell Receptor (BCR) Immune Repertoires with Abalign.Curr Protoc. 2024 Feb;4(2):e1002. doi: 10.1002/cpz1.1002. Curr Protoc. 2024. PMID: 38406972
-
B-cell receptor repertoire sequencing in patients with primary immunodeficiency: a review.Immunology. 2018 Feb;153(2):145-160. doi: 10.1111/imm.12865. Epub 2017 Dec 18. Immunology. 2018. PMID: 29140551 Free PMC article. Review.
-
B-cell repertoire dynamics after sequential hepatitis B vaccination and evidence for cross-reactive B-cell activation.Genome Med. 2016 Jun 16;8(1):68. doi: 10.1186/s13073-016-0322-z. Genome Med. 2016. PMID: 27312086 Free PMC article.
Cited by
-
Exploiting human immune repertoire transgenic mice for protective monoclonal antibodies against antimicrobial resistant Acinetobacter baumannii.Nat Commun. 2024 Sep 12;15(1):7979. doi: 10.1038/s41467-024-52357-8. Nat Commun. 2024. PMID: 39266557 Free PMC article.
-
Large-scale antibody immune response mapping of splenic B cells and bone marrow plasma cells in a transgenic mouse model.Front Immunol. 2023 Jun 5;14:1137069. doi: 10.3389/fimmu.2023.1137069. eCollection 2023. Front Immunol. 2023. PMID: 37346047 Free PMC article.
-
Biophysical cartography of the native and human-engineered antibody landscapes quantifies the plasticity of antibody developability.Commun Biol. 2024 Jul 31;7(1):922. doi: 10.1038/s42003-024-06561-3. Commun Biol. 2024. PMID: 39085379 Free PMC article.
-
Potent neutralization of SARS-CoV-2 variants by RBD nanoparticle and prefusion-stabilized spike immunogens.NPJ Vaccines. 2024 Oct 8;9(1):184. doi: 10.1038/s41541-024-00982-1. NPJ Vaccines. 2024. PMID: 39379400 Free PMC article.
-
Establishing RTS,S/AS01 as a benchmark for comparison to next-generation malaria vaccines in a mouse model.NPJ Vaccines. 2024 Feb 10;9(1):29. doi: 10.1038/s41541-024-00819-x. NPJ Vaccines. 2024. PMID: 38341502 Free PMC article.
References
Publication types
MeSH terms
Substances
Grants and funding
LinkOut - more resources
Full Text Sources