The cell cycle and cell size influence the rates of global cellular translation and transcription in fission yeast
- PMID: 36951016
- PMCID: PMC10152140
- DOI: 10.15252/embj.2022113333
The cell cycle and cell size influence the rates of global cellular translation and transcription in fission yeast
Abstract
How the production of biomass is controlled as cells increase in size and proceed through the cell cycle events is important for understanding the regulation of global cellular growth. This has been studied for decades but has not yielded consistent results, probably due to perturbations induced by the synchronisation methods used in most previous studies. To avoid this problem, we have developed a system to analyse unperturbed exponentially growing populations of fission yeast cells. We generated thousands of fixed single-cell measurements of cell size, cell cycle stage and the levels of global cellular translation and transcription. We show that translation scales with size, and additionally, increases at late S-phase/early G2 and early in mitosis and decreases later in mitosis, suggesting that cell cycle controls are also operative over global cellular translation. Transcription increases with both size and the amount of DNA, suggesting that the level of transcription of a cell may be the result of a dynamic equilibrium between the number of RNA polymerases associating and disassociating from DNA.
Keywords: cell cycle; cell size; scaling; transcription; translation.
© 2023 The Francis Crick Institute. Published under the terms of the CC BY 4.0 license.
Conflict of interest statement
All authors declare that they have no conflict of interest. Paul Nurse is a member of the Advisory Editorial Board of The EMBO Journal. This has no bearing on the editorial consideration of this article for publication.
Figures
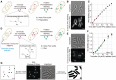
- A
Overview of the global cellular translation assay. Wild‐type cells are incubated with HPG for 5 min, then fixed, permeabilised and an Alexa Fluor azide fluorophore is covalently attached to HPG molecules using Click Chemistry.
- B
Example images of bright‐field and fluorescently labelled HPG (Alexa Fluor 647) of wild‐type cells (PN1) assayed for global cellular translation. Scale bars represent 5 μm.
- C
Change in HPG labelling signal with different durations of HPG incubation (in PN1), measured by flow cytometry. Population medians of at least 200,000 cells are shown. The red line is the ordinary least square (OLS) linear regression fitted on the medians between 0 and 5 min.
- D
Overview of the global cellular transcription assay. Cells expressing hENT1 and hsvTK are incubated with EU for 10 min, then fixed, permeabilised and an Alexa Fluor azide fluorophore is covalently attached to EU molecules using Click Chemistry.
- E
Example images of bright‐field and fluorescently labelled EU (Alexa Fluor 488) of hENT1 and hsvTK cells (PN10567) assayed for global cellular transcription. Scale bars represent 5 μm.
- F
Change in EU labelling signal with different lengths of EU incubations, measured by flow cytometry (in PN10597). The mean and standard deviation (SD) of the population medians of at least 200,000 cells in experimental triplicates are shown in black. The dark green line is the OLS linear regression fitted on the mean data between 2 and 12 min.
- G
Example images of bright field (PN10597) used to generate cell masks, and DNA (Nuclear‐ID Blue) used to generate nuclear masks. Scale bars represent 5 μm.
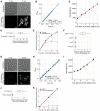
- A
Same as Fig 1B with time 0 included. The scale bar represents 5 μm.
- B
Growth curves of PN1 in EMM with or without 10 μM HPG at 25°C as measured by the change in optical density at 595 nm (OD595) over time. The doubling time of each condition indicated in the legend is calculated using the slope of the OLS linear regression fitted on the data after a natural logarithmic transformation.
- C
HPG signal shown in Fig 1C before normalisation and background removal.
- D
Wild‐type cells (PN1) were incubated for 30 min with 10 μM HPG, then assayed for global translation, treated with 0.05 mg/ml proteinase K at 55°C for 4 h and fluorescence was measured using flow cytometry. The 0.05, 0.25, 0.5, 0.75 and 0.95 population quantiles of at least 200,000 cells are shown.
- E
Wild‐type cells (PN1) were spun down and resuspended in EMM (control) or EMM + 10 mg/ml cycloheximide (t = 0), then assayed for global translation at different times using flow cytometry. Population medians of at least 200,000 cells are shown.
- F
Cells expressing hENT1 (PN6002), hsvTK (PN6003) or both (PN10597) were pulsed with 10 μM EU for 30 min and assayed for global transcription using flow cytometry. The 0.05, 0.25, 0.5, 0.75 and 0.95 population quantiles of at least 200,000 cells are shown.
- G
Same as Fig 1E with time 0 included. The scale bar represents 5 μm.
- H
Same as (B) for PN10597 grown with or without 20 μM EU.
- I
EU signal shown in Fig 1F before normalisation and background removal.
- J
Cells expressing hENT1 and hsvTK (PN10597) were pulsed with 10 μM EU and labelled with Alexa Fluor 488 azide, then treated with 0.1 mg/ml RNase A at 37°C for 16 h, and the fluorescence signal was assessed using flow cytometry. The 0.05, 0.25, 0.5, 0.75 and 0.95 population quantiles of at least 200,000 cells are shown.
- K
Cells expressing hENT1 and hsvTK (PN10597) were pulsed with EU plus DMSO or EU plus 300 μg/ml 1,10‐phenanthroline, and global transcription was assayed at different time intervals using flow cytometry. Population medians of at least 200,000 cells are shown.
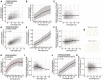
- A
Global cellular translation of wild‐type (PN1) single cells.
- B
Medians of global translation (solid black line) and interquartile ranges (IQR, shaded area) of cells shown in (A) grouped in length bins of 1 μm. Bins containing more than 50 cells are shown.
- C
Global cellular translation of cells shown in (A) divided by their cell length. The solid black line represents the OLS linear regression fitted on the data.
- D
Global cellular transcription of single cells expressing hENT1 and hsvTK (PN10597).
- E
Medians of global transcription (solid black line) and IQR (shaded area) of cells shown in (D) grouped in length bins of 1 μm. Bins containing more than 200 cells are shown.
- F
Global cellular transcription of cells shown in (D) divided by their cell length. The solid black line represents the OLS linear regression fitted on the data.
- G
Schematic representation of cell length in asynchronous populations of the wild type and the cdc25‐22 mutant grown at the semi‐permissive temperature (30°C).
- H
Medians of global translation (solid black line) and IQR (shaded area) of cdc25‐22 (PN143) cells grown at 30°C and grouped in length bins of 2 μm. Bins containing more than 30 cells are shown. The solid red lines represent a locally estimated scatterplot smoothing (LOESS) function fitted on the single‐cell data.
- I
Global cellular translation of cells shown in (H) divided by their cell length. The solid black line represents the LOESS function fitted in (H).
- J
Medians of global cellular transcription (solid black line) and IQR (shaded area) of cdc25‐22 hENT1 hsvTK (PN5998) cells grown at 30°C and grouped in length bins of 2 μm. Bins containing more than 100 cells are shown.
- K
Global cellular transcription of cells shown in (J) divided by their cell length. The solid black line represents the OLS linear regression fitted on the data.
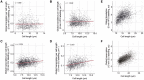
- A, B
Experimental replicates of Fig 2C.
- C, D
Experimental replicates of Fig 2F.
- E
Global cellular translation of cdc25‐22 (PN143) single cells.
- F
Global cellular transcription of hENT1 hsvTK cdc25‐22 (PN5998) single cells.
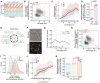
- A
Schematic representation of the G1, S and G2 subpopulation with overlapping cell sizes in the CCP∆ strain.
- B
CCP∆ (PN5792) cells were assayed for global cellular translation. The maximum DNA concentration, measured as the maximum fluorescence intensity of the Nuclear‐ID Blue stain in a cell, and cell length are used to categorise cells as having either 1C (blue box) or 2C DNA (red box). The percentage of cells in each category is shown.
- C
Cells shown in (B) are grouped in length bins of 1 μm. Medians of global cellular translation (solid lines) and IQR (shaded areas) are shown for 1C (blue) and 2C DNA (red) subpopulations. The dashed line box marks the length bins which have both a 1C and a 2C median global cellular translation values. Bins containing more than 50 cells are shown.
- D
For each of the five length bins boxed in (C), both 1C and 2C medians are normalised to their respective 1C global cellular translation values. The normalised values are represented as dots, each dot corresponding to one of the five length bins, the mean and SD of the normalised values are shown for each DNA content. For each DNA content, the normalised values (dots) are in the same order (left to right) as their corresponding length bins in (C). The P‐value is calculated using Welch's unequal variances t‐test.
- E
Schematic of EGFP‐PCNA localisation through the cell cycle.
- F
Example images of bright field and EGFP‐PCNA fluorescence in CCP∆ EGFP‐pcn1 cells (PN6001). The dashed lines in the EGFP‐PCNA channel delimit the cell masks generated from the bright‐field image. Cells with visible foci in the EGFP‐PCNA channel are highlighted in yellow and marked with arrows. Scale bars represent 5 μm.
- G
CCP∆ EGFP‐pcn1 cells (PN6001) were assayed for global cellular translation using a 5‐min HPG incubation. Cells with EGFP‐PCNA foci were identified by eye and binned in 1 μm intervals to compute the fraction of cells in S‐phase per cell length.
- H
The maximum local DNA concentration (the maximum fluorescence intensity of the Nuclear‐ID Blue DNA stain within a cell) and cell length were used to categorise cells not identified as in S‐phase in (G) has either in G1 (blue box) or G2/M (red box). The percentage of cells in each category is shown.
- I
Distribution of total DNA content of the cell populations categorised in (G) and (H) as measured per total Nuclear‐ID Blue fluorescence intensity per cell.
- J
Cells shown in (G) and (H) are grouped in length bins of 1 μm. Medians of global cellular translation (solid lines) and IQR (shaded areas) are shown for G1 (blue), S (yellow) and G2/M (red) subpopulations. The dashed line box marks the length bins which have G1, S and G2/M median global cellular translation values. Bins containing more than 75 cells are shown.
- K
For each of the four length bins boxed in (J), G1, S and G2/M medians are normalised to their respective G1 global cellular translation value. The normalised values are represented as dots, each dot corresponding to one of the four length bins, and the mean and SD of the normalised values are shown for each cell cycle stage. For each cell cycle stage, the normalised values (dots) are in the same order (left to right) as their corresponding length bins in (J). The P‐value is calculated using Welch's unequal variances t‐test.
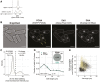
- A
Distribution of the amount of DNA in single cells in asynchronous populations, measured using the total fluorescence signal of Sytox Green by flow cytometry. For both populations, more than 200,000 cells were measured. Note that the 2C peak of the CCP∆ population (PN5999) is shifted to the right because the cells are longer and therefore have more mitochondrial DNA than the non‐delete strain (PN10597).
- B
hENT1 hsvTK EGFP‐pcn1 CCP∆ cells (PN6000) were pulsed with 200 μM EdU for 20 min and EdU incorporated in replicated DNA was fluorescently labelled using the same staining procedure used in the global transcription assay. Cells with visible foci in the PCNA channel are highlighted and marked with yellow arrows. The dotted white lines in the PCNA, EdU and DNA channels delimit the cell masks generated from the bright‐field image. The scale bar represents 5 μm.
- C
Distribution of maximum fluorescence intensity of cells labelled with EdU. The dashed line represents the threshold (3.25 au) above which cells are considered in S‐phase.
- D
The fraction of cells in S‐phase per cell length is computed using the EdU signal shown in (C), or the presence of EGFP‐PCNA foci determined by eye. The inset shows the overlap in cell numbers between the two methods of identifying S‐phase cells.
- E
Same as Fig 3H with the S‐phase population added in yellow.
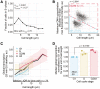
- A
hENT1 hsvTK CCP∆ EGFP‐pcn1 cells (PN6000) were assayed for global transcription using a 20‐min (almost linear, 15% deviation from the OLS linear regression shown in Fig 1F) EU incubation. Cells with EGFP‐PCNA foci were identified by eye and binned on 1 μm intervals to compute the fraction of cells in S‐phase per cell length.
- B
The maximum DNA concentration and cell length were used to categorise cells not identified as in S‐phase in (A) as either in G1 (blue box) or G2/M (red box). The percentage of cells in each category is shown.
- C
Cells shown in (A) and (B) are grouped in length bins of 1 μm. Medians of global cellular transcription (solid lines) and IQR (shaded areas) are shown for G1 (blue), S (yellow) and G2/M (red) subpopulations. The dashed line box marks the length bins which have G1, S and G2/M median global transcription values. Bins containing more than 75 cells are shown.
- D
For each of the three length bins boxed in (C), G1, S and G2/M medians are normalised to their respective G1 global transcription value. The normalised values are represented as dots, each dot corresponding to one of the three length bins, and the mean and SD of the normalised values are shown for each cell cycle stage. For each cell cycle stage, the normalised values (dots) are in the same order (left to right) as their corresponding length bins in (C). The P‐value is calculated using Welch's unequal variances t‐test.
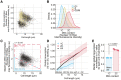
- A
Same as Fig 4B with the S‐phase population added in yellow.
- B
Distribution of total DNA content of the cell populations categorised in Fig 4A and B as measured per total Nuclear‐ID Blue fluorescence intensity per cell.
- C
hENT1 hsvTK CCP∆ (PN5999) cells were assayed for global transcription. The DNA concentration, measured as the maximum fluorescence intensity of the Nuclear‐ID Blue stain in a cell, and cell length are used to categorise cells as having either 1C (blue box) or 2C DNA (red box). The percentage of cells in each box is shown. Black dots are single‐cell measurements.
- D
Cells are grouped in bins of 1 μm. Medians (solid lines) and interquartile ranges (shaded areas) are shown for 1C (blue) or 2C DNA (red) populations. The dashed line box marks the length bins which have both a 1C and 2C median global transcription values.
- E
For each of the five length bins boxed in (B), both 1C and 2C medians are normalised to their respective global transcription 1C value. The normalised values are represented as dots, each dot corresponding to one of the five length bins, and the mean and SD of the normalised values are shown for each DNA content. The dots represent the median global transcription measurements per length bin boxed in (B). For each cell cycle stage, the normalised values (dots) are in the same order (left to right) as their corresponding length bins in (B). The P‐value is calculated using Welch's unequal variances t‐test.
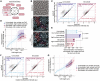
- A
Schematic of synCut3‐mCherry localisation through the cell cycle.
- B
Example images of bright field, synCut3‐mCherry, DNA (Nuclear‐ID Blue) fluorescence in synCut3‐mCherry cells (PN6004). The solid lines in the synCut3‐mCherry and DNA channels delimit the cell masks generated from the bright‐field image and are coloured according to the classification used in (D). The outlines of uninucleates are blue, binucleates are red, cells with high‐nuclear synCut3‐mCherry have dark outlines and cells with low‐nuclear synCut3‐mCherry have light outlines. Scale bars represent 5 μm.
- C
A population of synCut3‐mCherry cells (PN6004) was assayed for global cellular translation. The mean and median whole‐cell fluorescence synCut3‐mCherry intensities of uninucleates and binucleates were used to categorise cells as having low‐/high‐nuclear synCut3‐mCherry. The lines represent the delimitation of the different categories and the percentage of cells from the total population in each category is shown.
- D
Cells shown in (C) are grouped in length bins of 1 μm. Medians of global cellular translation (solid lines) and IQR (shaded areas) are shown for uninucleates (blue) and binucleates (red), and with low (light) and high (dark) nuclear synCut3‐mCherry signal subpopulations. The dashed line box marks the length bins which have median global cellular translation values for all four subpopulations. Bins containing more than 25 cells are shown.
- E
For each of the three length bins boxed in (D), the medians of each subpopulation are normalised to their respective “uninucleate, low nuclear synCut3‐mCherry” global cellular translation value. The normalised values are represented as dots, each dot corresponding to one of the three length bins, and the mean and SD of the normalised values are shown for each cell cycle stage. For each mitotic stage, the normalised values (dots) are in the same order (left to right) as their corresponding length bins in (D). The P‐value is calculated using Welch's unequal variances t‐test. For visual clarity, synCut3‐mCherry is shortened to synCut3 in fig.
- F
Same as (C) for synCut3‐mCherry hENT1 hsvTK cells (PN6005) assayed for global transcription.
- G
Cells shown in (F) are grouped in length bins of 1 μm. Medians of global cellular transcription (solid lines) and IQR (shaded areas) are shown for uninucleates (blue) and binucleates (red), and with low‐ (light) and high (dark)‐nuclear synCut3‐mCherry signal subpopulations. Bins containing more than 25 cells are shown.
Similar articles
-
Regulation of global translation during the cell cycle.J Cell Sci. 2018 Sep 3;131(17):jcs220327. doi: 10.1242/jcs.220327. J Cell Sci. 2018. PMID: 30072440
-
How do fission yeast cells grow and connect growth to the mitotic cycle?Curr Genet. 2017 May;63(2):165-173. doi: 10.1007/s00294-016-0632-0. Epub 2016 Jul 27. Curr Genet. 2017. PMID: 27465359 Review.
-
The chk1 pathway is required to prevent mitosis following cell-cycle arrest at 'start'.Curr Biol. 1995 Oct 1;5(10):1179-90. doi: 10.1016/s0960-9822(95)00234-x. Curr Biol. 1995. PMID: 8548290
-
Identification of mutants with increased variation in cell size at onset of mitosis in fission yeast.J Cell Sci. 2021 Feb 11;134(3):jcs251769. doi: 10.1242/jcs.251769. J Cell Sci. 2021. PMID: 33419777 Free PMC article.
-
Cell cycle molecules and mechanisms of the budding and fission yeasts.Methods Mol Biol. 2005;296:3-29. doi: 10.1385/1-59259-857-9:003. Methods Mol Biol. 2005. PMID: 15576924 Review.
Cited by
-
Gene expression in growing cells: A biophysical primer.ArXiv [Preprint]. 2023 Nov 20:arXiv:2311.12143v1. ArXiv. 2023. PMID: 38045483 Free PMC article. Preprint.
-
Constant surface area-to-volume ratio during cell growth as a design principle in mammalian cells.bioRxiv [Preprint]. 2024 Jul 18:2024.07.02.601447. doi: 10.1101/2024.07.02.601447. bioRxiv. 2024. PMID: 39005340 Free PMC article. Preprint.
-
The antiproliferative effect of FGF2 in K-Ras-driven tumor cells involves modulation of rRNA and the nucleolus.J Cell Sci. 2023 Nov 15;136(22):jcs260989. doi: 10.1242/jcs.260989. Epub 2023 Nov 30. J Cell Sci. 2023. PMID: 37921359 Free PMC article.
-
Stochastic Gene Expression in Proliferating Cells: Differing Noise Intensity in Single-Cell and Population Perspectives.bioRxiv [Preprint]. 2024 Jun 29:2024.06.28.601263. doi: 10.1101/2024.06.28.601263. bioRxiv. 2024. PMID: 38979195 Free PMC article. Preprint.
-
Cytoplasmic fluidization contributes to breaking spore dormancy in fission yeast.Proc Natl Acad Sci U S A. 2024 Jun 25;121(26):e2405553121. doi: 10.1073/pnas.2405553121. Epub 2024 Jun 18. Proc Natl Acad Sci U S A. 2024. PMID: 38889144 Free PMC article.
References
-
- Berg S, Kutra D, Kroeger T, Straehle CN, Kausler BX, Haubold C, Schiegg M, Ales J, Beier T, Rudy M et al (2019) Ilastik: interactive machine learning for (bio)image analysis. Nat Methods 16: 1226–1232 - PubMed
-
- Berry S, Müller M, Rai A, Pelkmans L (2022) Feedback from nuclear RNA on transcription promotes robust RNA concentration homeostasis in human cells. Cell Syst 13: 454–470.e15 - PubMed
-
- Cheng L, Chen J, Kong Y, Tan C, Kafri R, Björklund M (2021) Size‐scaling promotes senescence‐like changes in proteome and organelle content. bioRxiv 10.1101/2021.08.05.455193 [PREPRINT] - DOI
Publication types
MeSH terms
Substances
Grants and funding
LinkOut - more resources
Full Text Sources