Immune evasion of neutralizing antibodies by SARS-CoV-2 Omicron
- PMID: 36948931
- PMCID: PMC9985919
- DOI: 10.1016/j.cytogfr.2023.03.001
Immune evasion of neutralizing antibodies by SARS-CoV-2 Omicron
Erratum in
-
Corrigendum to "Immune evasion of neutralizing antibodies by SARS-CoV-2 Omicron" [Cytokine Growth Factor Rev. 70 (2023) 13-25].Cytokine Growth Factor Rev. 2024 Jun;77:117. doi: 10.1016/j.cytogfr.2024.03.006. Epub 2024 Apr 13. Cytokine Growth Factor Rev. 2024. PMID: 38614914 Free PMC article. No abstract available.
Abstract
Since its emergence at the end of 2019, severe acute respiratory syndrome coronavirus 2 (SARS-CoV-2) has caused the infection of more than 600 million people worldwide and has significant damage to global medical, economic, and political structures. Currently, a highly mutated variant of concern, SARS-CoV-2 Omicron, has evolved into many different subvariants mainly including BA.1, BA.2, BA.3, BA.4/5, and the recently emerging BA.2.75.2, BA.2.76, BA.4.6, BA.4.7, BA.5.9, BF.7, BQ.1, BQ.1.1, XBB, XBB.1, etc. Mutations in the N-terminal domain (NTD) of the spike protein, such as A67V, G142D, and N212I, alter the antigenic structure of Omicron, while mutations in the spike receptor binding domain (RBD), such as R346K, Q493R, and N501Y, increase the affinity for angiotensin-converting enzyme 2 (ACE2). Both types of mutations greatly increase the capacity of Omicron to evade immunity from neutralizing antibodies, produced by natural infection and/or vaccination. In this review, we systematically assess the immune evasion capacity of SARS-CoV-2, with an emphasis on the neutralizing antibodies generated by different vaccination regimes. Understanding the host antibody response and the evasion strategies employed by SARS-CoV-2 variants will improve our capacity to combat newly emerging Omicron variants.
Keywords: Bivalent mRNA vaccine; Booster; Immune evasion; Mutations; Omicron; SARS-CoV-2.
Copyright © 2023 Elsevier Ltd. All rights reserved.
Conflict of interest statement
Conflict of interest The authors declare that they have no conflict of interest.
Figures
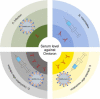
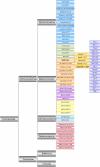
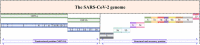
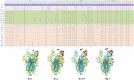
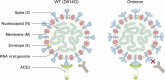
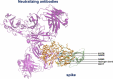
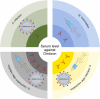
Similar articles
-
Potent antibodies against immune invasive SARS-CoV-2 Omicron subvariants.Int J Biol Macromol. 2023 Sep 30;249:125997. doi: 10.1016/j.ijbiomac.2023.125997. Epub 2023 Jul 26. Int J Biol Macromol. 2023. PMID: 37499711 Review.
-
Characterization of Entry Pathways, Species-Specific Angiotensin-Converting Enzyme 2 Residues Determining Entry, and Antibody Neutralization Evasion of Omicron BA.1, BA.1.1, BA.2, and BA.3 Variants.J Virol. 2022 Sep 14;96(17):e0114022. doi: 10.1128/jvi.01140-22. Epub 2022 Aug 24. J Virol. 2022. PMID: 36000843 Free PMC article.
-
Temperature-dependent Spike-ACE2 interaction of Omicron subvariants is associated with viral transmission.mBio. 2024 Aug 14;15(8):e0090724. doi: 10.1128/mbio.00907-24. Epub 2024 Jul 2. mBio. 2024. PMID: 38953636 Free PMC article.
-
The Biological Functions and Clinical Significance of SARS-CoV-2 Variants of Corcern.Front Med (Lausanne). 2022 May 20;9:849217. doi: 10.3389/fmed.2022.849217. eCollection 2022. Front Med (Lausanne). 2022. PMID: 35669924 Free PMC article. Review.
-
Cross-neutralizing antibody against emerging Omicron subvariants of SARS-CoV-2 in infection-naïve individuals with homologous BNT162b2 or BNT162b2(WT + BA.4/5) bivalent booster vaccination.Virol J. 2024 Mar 21;21(1):70. doi: 10.1186/s12985-024-02335-9. Virol J. 2024. PMID: 38515117 Free PMC article.
Cited by
-
Exigency of Plant-Based Vaccine against COVID-19 Emergence as Pandemic Preparedness.Vaccines (Basel). 2023 Aug 9;11(8):1347. doi: 10.3390/vaccines11081347. Vaccines (Basel). 2023. PMID: 37631915 Free PMC article. Review.
-
Comparative Analysis of Vaccine-Induced Neutralizing Antibodies against the Alpha, Beta, Delta, and Omicron Variants of SARS-CoV-2.Vaccines (Basel). 2024 May 9;12(5):515. doi: 10.3390/vaccines12050515. Vaccines (Basel). 2024. PMID: 38793766 Free PMC article.
-
The Promotion of Humoral Immune Responses in Humans via SOCS1-Mediated Th2-Bias Following SARS-CoV-2 Vaccination.Vaccines (Basel). 2023 Nov 20;11(11):1730. doi: 10.3390/vaccines11111730. Vaccines (Basel). 2023. PMID: 38006062 Free PMC article.
-
Comparative analysis of clinical and immunological profiles across Omicron BA.5.2 subvariants using next-generation sequencing in a Chinese cohort.Front Cell Infect Microbiol. 2023 Oct 30;13:1288914. doi: 10.3389/fcimb.2023.1288914. eCollection 2023. Front Cell Infect Microbiol. 2023. PMID: 37965255 Free PMC article.
-
Secure reversal of immune evasion from refractory NSCLC and highly contagious CoV-2 mutants by using 3D-engineered multifunctional biologics.Bioeng Transl Med. 2023 Jun 24;8(5):e10554. doi: 10.1002/btm2.10554. eCollection 2023 Sep. Bioeng Transl Med. 2023. PMID: 37693048 Free PMC article.
References
Publication types
MeSH terms
Substances
Supplementary concepts
LinkOut - more resources
Full Text Sources
Medical
Miscellaneous