Human Neuromuscular Junction on a Chip: Impact of Amniotic Fluid Stem Cell Extracellular Vesicles on Muscle Atrophy and NMJ Integrity
- PMID: 36902375
- PMCID: PMC10003237
- DOI: 10.3390/ijms24054944
Human Neuromuscular Junction on a Chip: Impact of Amniotic Fluid Stem Cell Extracellular Vesicles on Muscle Atrophy and NMJ Integrity
Abstract
Neuromuscular junctions (NMJs) are specialized synapses, crucial for the communication between spinal motor neurons (MNs) and skeletal muscle. NMJs become vulnerable in degenerative diseases, such as muscle atrophy, where the crosstalk between the different cell populations fails, and the regenerative ability of the entire tissue is hampered. How skeletal muscle sends retrograde signals to MNs through NMJs represents an intriguing field of research, and the role of oxidative stress and its sources remain poorly understood. Recent works demonstrate the myofiber regeneration potential of stem cells, including amniotic fluid stem cells (AFSC), and secreted extracellular vesicles (EVs) as cell-free therapy. To study NMJ perturbations during muscle atrophy, we generated an MN/myotube co-culture system through XonaTM microfluidic devices, and muscle atrophy was induced in vitro by Dexamethasone (Dexa). After atrophy induction, we treated muscle and MN compartments with AFSC-derived EVs (AFSC-EVs) to investigate their regenerative and anti-oxidative potential in counteracting NMJ alterations. We found that the presence of EVs reduced morphological and functional in vitro defects induced by Dexa. Interestingly, oxidative stress, occurring in atrophic myotubes and thus involving neurites as well, was prevented by EV treatment. Here, we provided and validated a fluidically isolated system represented by microfluidic devices for studying human MN and myotube interactions in healthy and Dexa-induced atrophic conditions-allowing the isolation of subcellular compartments for region-specific analyses-and demonstrated the efficacy of AFSC-EVs in counteracting NMJ perturbations.
Keywords: amniotic fluid stem cells; extracellular vesicles; muscle atrophy; neuromuscular junction; oxidative stress.
Conflict of interest statement
The authors declare no conflict of interest.
Figures
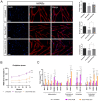
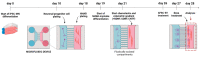
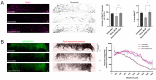
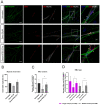
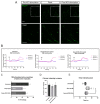
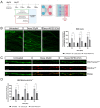
Similar articles
-
Immersed in a reservoir of potential: amniotic fluid-derived extracellular vesicles.J Transl Med. 2024 Apr 12;22(1):348. doi: 10.1186/s12967-024-05154-2. J Transl Med. 2024. PMID: 38609955 Free PMC article. Review.
-
The Regenerative Potential of Amniotic Fluid Stem Cell Extracellular Vesicles: Lessons Learned by Comparing Different Isolation Techniques.Sci Rep. 2019 Feb 12;9(1):1837. doi: 10.1038/s41598-018-38320-w. Sci Rep. 2019. PMID: 30755672 Free PMC article.
-
Generation of Human Motor Units with Functional Neuromuscular Junctions in Microfluidic Devices.J Vis Exp. 2021 Sep 7;(175). doi: 10.3791/62959. J Vis Exp. 2021. PMID: 34570099
-
Extracellular vesicles from II trimester human amniotic fluid as paracrine conveyors counteracting oxidative stress.Redox Biol. 2024 Sep;75:103241. doi: 10.1016/j.redox.2024.103241. Epub 2024 Jun 17. Redox Biol. 2024. PMID: 38901103 Free PMC article.
-
How to Build and to Protect the Neuromuscular Junction: The Role of the Glial Cell Line-Derived Neurotrophic Factor.Int J Mol Sci. 2020 Dec 24;22(1):136. doi: 10.3390/ijms22010136. Int J Mol Sci. 2020. PMID: 33374485 Free PMC article. Review.
Cited by
-
Immersed in a reservoir of potential: amniotic fluid-derived extracellular vesicles.J Transl Med. 2024 Apr 12;22(1):348. doi: 10.1186/s12967-024-05154-2. J Transl Med. 2024. PMID: 38609955 Free PMC article. Review.
-
Resveratrol and Vitamin D: Eclectic Molecules Promoting Mitochondrial Health in Sarcopenia.Int J Mol Sci. 2024 Jul 9;25(14):7503. doi: 10.3390/ijms25147503. Int J Mol Sci. 2024. PMID: 39062745 Free PMC article. Review.
-
Unraveling the causes of sarcopenia: Roles of neuromuscular junction impairment and mitochondrial dysfunction.Physiol Rep. 2024 Jan;12(1):e15917. doi: 10.14814/phy2.15917. Physiol Rep. 2024. PMID: 38225199 Free PMC article. Review.
References
MeSH terms
Grants and funding
LinkOut - more resources
Full Text Sources