3D-printed fish gelatin scaffolds for cartilage tissue engineering
- PMID: 36875052
- PMCID: PMC9974427
- DOI: 10.1016/j.bioactmat.2023.02.007
3D-printed fish gelatin scaffolds for cartilage tissue engineering
Abstract
Knee osteoarthritis is a chronic disease caused by the deterioration of the knee joint due to various factors such as aging, trauma, and obesity, and the nonrenewable nature of the injured cartilage makes the treatment of osteoarthritis challenging. Here, we present a three-dimensional (3D) printed porous multilayer scaffold based on cold-water fish skin gelatin for osteoarticular cartilage regeneration. To make the scaffold, cold-water fish skin gelatin was combined with sodium alginate to increase viscosity, printability, and mechanical strength, and the hybrid hydrogel was printed according to a pre-designed specific structure using 3D printing technology. Then, the printed scaffolds underwent a double-crosslinking process to enhance their mechanical strength even further. These scaffolds mimic the structure of the original cartilage network in a way that allows chondrocytes to adhere, proliferate, and communicate with each other, transport nutrients, and prevent further damage to the joint. More importantly, we found that cold-water fish gelatin scaffolds were nonimmunogenic, nontoxic, and biodegradable. We also implanted the scaffold into defective rat cartilage for 12 weeks and achieved satisfactory repair results in this animal model. Thus, cold-water fish skin gelatin scaffolds may have broad application potential in regenerative medicine.
Keywords: 3D printing; Cartilage defect repair; Fish skin gelatin; Sodium alginate; Tissue engineering.
© 2023 The Authors.
Conflict of interest statement
The authors declare no competing financial interests and agreed to author contributions statements below.
Figures
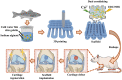
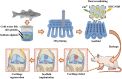
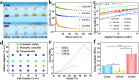
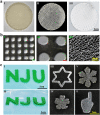
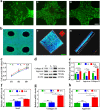
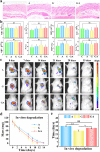
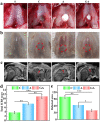
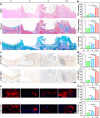
Similar articles
-
3D Printed Chitosan Composite Scaffold for Chondrocytes Differentiation.Curr Med Imaging. 2021;17(7):832-842. doi: 10.2174/1573405616666201217112939. Curr Med Imaging. 2021. PMID: 33334294
-
Lyophilized Scaffolds Fabricated from 3D-Printed Photocurable Natural Hydrogel for Cartilage Regeneration.ACS Appl Mater Interfaces. 2018 Sep 19;10(37):31704-31715. doi: 10.1021/acsami.8b10926. Epub 2018 Sep 10. ACS Appl Mater Interfaces. 2018. PMID: 30157627
-
Dual-crosslinked 3D printed gelatin scaffolds with potential for temporomandibular joint cartilage regeneration.Biomed Mater. 2021 Mar 5;16(3). doi: 10.1088/1748-605X/abe6d9. Biomed Mater. 2021. PMID: 33592589
-
Biomolecules-Loading of 3D-Printed Alginate-Based Scaffolds for Cartilage Tissue Engineering Applications: A Review on Current Status and Future Prospective.Arch Bone Jt Surg. 2024;12(2):92-101. doi: 10.22038/ABJS.2023.73275.3396. Arch Bone Jt Surg. 2024. PMID: 38420521 Free PMC article. Review.
-
Toward Biomimetic Scaffolds for Tissue Engineering: 3D Printing Techniques in Regenerative Medicine.Front Bioeng Biotechnol. 2020 Nov 4;8:586406. doi: 10.3389/fbioe.2020.586406. eCollection 2020. Front Bioeng Biotechnol. 2020. PMID: 33251199 Free PMC article. Review.
Cited by
-
3D printing of Ceffe-infused scaffolds for tailored nipple-like cartilage development.BMC Biotechnol. 2024 Apr 30;24(1):25. doi: 10.1186/s12896-024-00848-3. BMC Biotechnol. 2024. PMID: 38689309 Free PMC article.
-
Decellularized Tumor Tissues Integrated with Polydopamine for Wound Healing.Research (Wash D C). 2024 Aug 6;7:0445. doi: 10.34133/research.0445. eCollection 2024. Research (Wash D C). 2024. PMID: 39109247 Free PMC article.
-
Advances in 3D printing for the repair of tympanic membrane perforation: a comprehensive review.Front Bioeng Biotechnol. 2024 Aug 12;12:1439499. doi: 10.3389/fbioe.2024.1439499. eCollection 2024. Front Bioeng Biotechnol. 2024. PMID: 39188376 Free PMC article. Review.
-
Raising the Bar: Progress in 3D-Printed Hybrid Bone Scaffolds for Clinical Applications: A Review.Cell Transplant. 2024 Jan-Dec;33:9636897241273562. doi: 10.1177/09636897241273562. Cell Transplant. 2024. PMID: 39517106 Free PMC article. Review.
-
The Effect of Nozzle Temperature on the Low-Temperature Printing Performance of Low-Viscosity Food Ink.Foods. 2023 Jul 11;12(14):2666. doi: 10.3390/foods12142666. Foods. 2023. PMID: 37509758 Free PMC article.
References
LinkOut - more resources
Full Text Sources