A change of heart: understanding the mechanisms regulating cardiac proliferation and metabolism before and after birth
- PMID: 36872609
- PMCID: PMC10952280
- DOI: 10.1113/JP284137
A change of heart: understanding the mechanisms regulating cardiac proliferation and metabolism before and after birth
Abstract
Mammalian cardiomyocytes undergo major maturational changes in preparation for birth and postnatal life. Immature cardiomyocytes contribute to cardiac growth via proliferation and thus the heart has the capacity to regenerate. To prepare for postnatal life, structural and metabolic changes associated with increased cardiac output and function must occur. This includes exit from the cell cycle, hypertrophic growth, mitochondrial maturation and sarcomeric protein isoform switching. However, these changes come at a price: the loss of cardiac regenerative capacity such that damage to the heart in postnatal life is permanent. This is a significant barrier to the development of new treatments for cardiac repair and contributes to heart failure. The transitional period of cardiomyocyte growth is a complex and multifaceted event. In this review, we focus on studies that have investigated this critical transition period as well as novel factors that may regulate and drive this process. We also discuss the potential use of new biomarkers for the detection of myocardial infarction and, in the broader sense, cardiovascular disease.
Keywords: biomarkers; fetal development; heart attack; heart disease; miRNA; programming; regeneration.
© 2023 The Authors. The Journal of Physiology published by John Wiley & Sons Ltd on behalf of The Physiological Society.
Conflict of interest statement
The authors have no conflicts of interest.
Figures
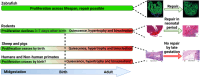
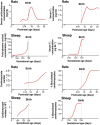
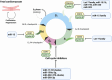
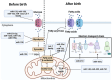
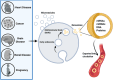
Similar articles
-
Transcriptional Regulation of Postnatal Cardiomyocyte Maturation and Regeneration.Int J Mol Sci. 2021 Mar 23;22(6):3288. doi: 10.3390/ijms22063288. Int J Mol Sci. 2021. PMID: 33807107 Free PMC article. Review.
-
RNA-Binding Protein LIN28a Regulates New Myocyte Formation in the Heart Through Long Noncoding RNA-H19.Circulation. 2023 Jan 24;147(4):324-337. doi: 10.1161/CIRCULATIONAHA.122.059346. Epub 2022 Oct 31. Circulation. 2023. PMID: 36314132 Free PMC article.
-
Prolonged Myocardial Regenerative Capacity in Neonatal Opossum.Circulation. 2022 Jul 12;146(2):125-139. doi: 10.1161/CIRCULATIONAHA.121.055269. Epub 2022 May 26. Circulation. 2022. PMID: 35616010
-
Multicellular Transcriptional Analysis of Mammalian Heart Regeneration.Circulation. 2017 Sep 19;136(12):1123-1139. doi: 10.1161/CIRCULATIONAHA.117.028252. Epub 2017 Jul 21. Circulation. 2017. PMID: 28733351 Free PMC article.
-
Cardiomyocyte maturation and its reversal during cardiac regeneration.Dev Dyn. 2024 Jan;253(1):8-27. doi: 10.1002/dvdy.557. Epub 2022 Dec 21. Dev Dyn. 2024. PMID: 36502296 Review.
Cited by
-
Insulin-Activated Signaling Pathway and GLUT4 Membrane Translocation in hiPSC-Derived Cardiomyocytes.Int J Mol Sci. 2024 Jul 27;25(15):8197. doi: 10.3390/ijms25158197. Int J Mol Sci. 2024. PMID: 39125765 Free PMC article.
-
Volume overload impedes the maturation of sarcomeres and T-tubules in the right atria: a potential cause of atrial arrhythmia following delayed atrial septal defect closure.Front Physiol. 2023 Oct 16;14:1237187. doi: 10.3389/fphys.2023.1237187. eCollection 2023. Front Physiol. 2023. PMID: 37908335 Free PMC article.
-
Relationship of Acylcarnitines to Myocardial Ischemic Remodeling and Clinical Manifestations in Chronic Heart Failure.J Cardiovasc Dev Dis. 2023 Oct 21;10(10):438. doi: 10.3390/jcdd10100438. J Cardiovasc Dev Dis. 2023. PMID: 37887885 Free PMC article.
-
Comparative Metabolomics in Single Ventricle Patients after Fontan Palliation: A Strong Case for a Targeted Metabolic Therapy.Metabolites. 2023 Aug 9;13(8):932. doi: 10.3390/metabo13080932. Metabolites. 2023. PMID: 37623876 Free PMC article. Review.
-
Maturation of lipid metabolism in the fetal and newborn sheep heart.Am J Physiol Regul Integr Comp Physiol. 2023 Dec 1;325(6):R809-R819. doi: 10.1152/ajpregu.00122.2023. Epub 2023 Oct 23. Am J Physiol Regul Integr Comp Physiol. 2023. PMID: 37867472 Free PMC article.
References
-
- Adler, C. P. , Friedburg, H. , Herget, G. W. , Neuburger, M. , & Schwalb, H. (1996). Variability of cardiomyocyte DNA content, ploidy level and nuclear number in mammalian hearts. Virchows Archiv: An International Journal of Pathology, 429(2–3), 159–164. - PubMed
-
- Agnew, E. J. , Velayutham, N. , Matos Ortiz, G. , Alfieri, C. M. , Hortells, L. , Moore, V. , Riggs, K. W. , Baker, R. S. , Gibson, A. M. , Ponny, S. R. , Alsaied, T. , Zafar, F. , & Yutzey, K. E. (2020). Scar formation with decreased cardiac function following ischemia/reperfusion injury in 1 month old swine. Journal of Cardiovascular Development and Disease, 7(1), 1. - PMC - PubMed
Publication types
MeSH terms
LinkOut - more resources
Full Text Sources
Medical