Flavivirus nonstructural proteins and replication complexes as antiviral drug targets
- PMID: 36870091
- PMCID: PMC10023477
- DOI: 10.1016/j.coviro.2023.101305
Flavivirus nonstructural proteins and replication complexes as antiviral drug targets
Abstract
Many flaviviruses are well-known pathogens, such as dengue, Zika, Japanese encephalitis, and yellow fever viruses. Among them, dengue viruses cause global epidemics and threaten billions of people. Effective vaccines and antivirals are in desperate need. In this review, we focus on the recent advances in understanding viral nonstructural (NS) proteins as antiviral drug targets. We briefly summarize the experimental structures and predicted models of flaviviral NS proteins and their functions. We highlight a few well-characterized inhibitors targeting these NS proteins and provide an update about the latest development. NS4B emerges as one of the most promising drug targets as novel inhibitors targeting NS4B and its interaction network are entering clinical studies. Studies aiming to elucidate the architecture and molecular basis of viral replication will offer new opportunities for novel antiviral discovery. Direct-acting agents against dengue and other pathogenic flaviviruses may be available very soon.
Copyright © 2023 Elsevier B.V. All rights reserved.
Conflict of interest statement
Conflict of interest statement No potential conflicts of interest were disclosed.
Figures
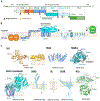
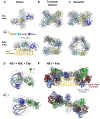
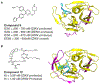
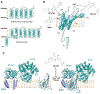
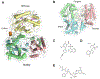
Similar articles
-
Small-Molecule Inhibitor of Flaviviral NS3-NS5 Interaction with Broad-Spectrum Activity and Efficacy In Vivo.mBio. 2023 Feb 28;14(1):e0309722. doi: 10.1128/mbio.03097-22. Epub 2023 Jan 9. mBio. 2023. PMID: 36622141 Free PMC article.
-
Flavivirus NS4B protein: Structure, function, and antiviral discovery.Antiviral Res. 2022 Nov;207:105423. doi: 10.1016/j.antiviral.2022.105423. Epub 2022 Sep 27. Antiviral Res. 2022. PMID: 36179934 Free PMC article. Review.
-
Flaviviral NS4b, chameleon and jack-in-the-box roles in viral replication and pathogenesis, and a molecular target for antiviral intervention.Rev Med Virol. 2015 Jul;25(4):205-23. doi: 10.1002/rmv.1835. Epub 2015 Apr 1. Rev Med Virol. 2015. PMID: 25828437 Free PMC article. Review.
-
Viperin Restricts Zika Virus and Tick-Borne Encephalitis Virus Replication by Targeting NS3 for Proteasomal Degradation.J Virol. 2018 Mar 14;92(7):e02054-17. doi: 10.1128/JVI.02054-17. Print 2018 Apr 1. J Virol. 2018. PMID: 29321318 Free PMC article.
-
Flavivirus enzymes and their inhibitors.Enzymes. 2021;49:265-303. doi: 10.1016/bs.enz.2021.07.006. Epub 2021 Sep 1. Enzymes. 2021. PMID: 34696835 Free PMC article.
Cited by
-
Recent advances in the study of zika virus structure, drug targets, and inhibitors.Front Pharmacol. 2024 Jul 1;15:1418516. doi: 10.3389/fphar.2024.1418516. eCollection 2024. Front Pharmacol. 2024. PMID: 39011504 Free PMC article. Review.
-
Structural Insights into the Dynamic Assembly of a YFV sNS1 Tetramer.Viruses. 2024 Jul 29;16(8):1212. doi: 10.3390/v16081212. Viruses. 2024. PMID: 39205186 Free PMC article.
-
Japanese Encephalitis: Risk of Emergence in the United States and the Resulting Impact.Viruses. 2023 Dec 28;16(1):54. doi: 10.3390/v16010054. Viruses. 2023. PMID: 38257754 Free PMC article.
-
Flavivirus Zika NS4A protein forms large oligomers in liposomes and in mild detergent.Sci Rep. 2024 May 31;14(1):12533. doi: 10.1038/s41598-024-63407-y. Sci Rep. 2024. PMID: 38822066 Free PMC article.
-
Orthoflaviviral Inhibitors in Clinical Trials, Preclinical In Vivo Efficacy Targeting NS2B-NS3 and Cellular Antiviral Activity via Competitive Protease Inhibition.Molecules. 2024 Aug 27;29(17):4047. doi: 10.3390/molecules29174047. Molecules. 2024. PMID: 39274895 Free PMC article. Review.
References
-
- Gyawali N, Bradbury RS, and Taylor-Robinson AW, The epidemiology of dengue infection: Harnessing past experience and current knowledge to support implementation of future control strategies. J Vector Borne Dis, 2016. 53(4): p. 293–304. - PubMed
-
- Solomon T and Vaughn DW, Pathogenesis and clinical features of Japanese encephalitis and West Nile virus infections. Curr Top Microbiol Immunol, 2002. 267: p. 171–94. - PubMed
-
- Pierson TC and Diamond MS, The emergence of Zika virus and its new clinical syndromes. Nature, 2018. 560(7720): p. 573–581. - PubMed
-
- WHO, Dengue guidelines, for diagnosis, treatment, prevention and control. 2009, World Health Organization: Geneva. p. 157. - PubMed
Publication types
MeSH terms
Substances
Grants and funding
LinkOut - more resources
Full Text Sources
Medical
Miscellaneous