DAXX adds a de novo H3.3K9me3 deposition pathway to the histone chaperone network
- PMID: 36868228
- PMCID: PMC10114496
- DOI: 10.1016/j.molcel.2023.02.009
DAXX adds a de novo H3.3K9me3 deposition pathway to the histone chaperone network
Abstract
A multitude of histone chaperones are required to support histones from their biosynthesis until DNA deposition. They cooperate through the formation of histone co-chaperone complexes, but the crosstalk between nucleosome assembly pathways remains enigmatic. Using exploratory interactomics, we define the interplay between human histone H3-H4 chaperones in the histone chaperone network. We identify previously uncharacterized histone-dependent complexes and predict the structure of the ASF1 and SPT2 co-chaperone complex, expanding the role of ASF1 in histone dynamics. We show that DAXX provides a unique functionality to the histone chaperone network, recruiting histone methyltransferases to promote H3K9me3 catalysis on new histone H3.3-H4 prior to deposition onto DNA. Hereby, DAXX provides a molecular mechanism for de novo H3K9me3 deposition and heterochromatin assembly. Collectively, our findings provide a framework for understanding how cells orchestrate histone supply and employ targeted deposition of modified histones to underpin specialized chromatin states.
Keywords: ASF1; DAXX; HJURP; NASP; epigenetic; gene silencing; heterochromatin; histone chaperone; nucleosome assembly; protein network; proteomics.
Copyright © 2023 The Author(s). Published by Elsevier Inc. All rights reserved.
Conflict of interest statement
Declaration of interests C.M.H. and A.G. are inventors on a patent covering the therapeutic targeting of TONSL for cancer therapy. A.G. is co-founder and chief scientific officer of Ankrin Therapeutics. A.G. is a member of Molecular Cell’s Scientific Advisory Board. A.I. and M.V.-A. are cofounders of EpiQMAx. G.M. is co-founder and member of the board of directors of Twelve Bio and is a member of the Scientific Advisory Board at Ensoma.
Figures
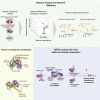
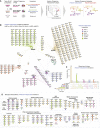
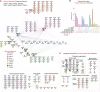
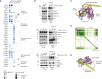
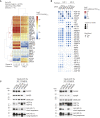
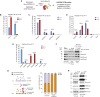
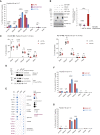
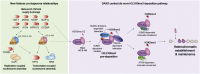
Comment in
-
Histone chaperones: A multinodal highway network inside the cell.Mol Cell. 2023 Apr 6;83(7):1024-1026. doi: 10.1016/j.molcel.2023.03.004. Mol Cell. 2023. PMID: 37028413 Free PMC article.
-
Histone H3 and its chaperones.Nat Struct Mol Biol. 2023 Apr;30(4):405. doi: 10.1038/s41594-023-00976-y. Nat Struct Mol Biol. 2023. PMID: 37045977 No abstract available.
Similar articles
-
PML is recruited to heterochromatin during S phase and represses DAXX-mediated histone H3.3 chromatin assembly.J Cell Sci. 2019 Mar 26;132(6):jcs220970. doi: 10.1242/jcs.220970. J Cell Sci. 2019. PMID: 30796101 Free PMC article.
-
H3.Y discriminates between HIRA and DAXX chaperone complexes and reveals unexpected insights into human DAXX-H3.3-H4 binding and deposition requirements.Nucleic Acids Res. 2017 Jun 2;45(10):5691-5706. doi: 10.1093/nar/gkx131. Nucleic Acids Res. 2017. PMID: 28334823 Free PMC article.
-
The death-associated protein DAXX is a novel histone chaperone involved in the replication-independent deposition of H3.3.Genes Dev. 2010 Jun 15;24(12):1253-65. doi: 10.1101/gad.566910. Epub 2010 May 26. Genes Dev. 2010. PMID: 20504901 Free PMC article.
-
The histone chaperone Asf1 at the crossroads of chromatin and DNA checkpoint pathways.Chromosoma. 2007 Apr;116(2):79-93. doi: 10.1007/s00412-006-0087-z. Epub 2006 Dec 19. Chromosoma. 2007. PMID: 17180700 Review.
-
Histone chaperones: 30 years from isolation to elucidation of the mechanisms of nucleosome assembly and disassembly.Cell Mol Life Sci. 2008 Feb;65(3):414-44. doi: 10.1007/s00018-007-7305-6. Cell Mol Life Sci. 2008. PMID: 17955179 Free PMC article. Review.
Cited by
-
Acute multi-level response to defective de novo chromatin assembly in S-phase.bioRxiv [Preprint]. 2024 Mar 27:2024.03.22.586291. doi: 10.1101/2024.03.22.586291. bioRxiv. 2024. Update in: Mol Cell. 2024 Dec 19;84(24):4711-4728.e10. doi: 10.1016/j.molcel.2024.10.023 PMID: 38585916 Free PMC article. Updated. Preprint.
-
HIRA vs. DAXX: the two axes shaping the histone H3.3 landscape.Exp Mol Med. 2024 Feb;56(2):251-263. doi: 10.1038/s12276-023-01145-3. Epub 2024 Feb 1. Exp Mol Med. 2024. PMID: 38297159 Free PMC article. Review.
-
Lysine-36 of Drosophila histone H3.3 supports adult longevity.bioRxiv [Preprint]. 2023 Dec 13:2023.09.28.559962. doi: 10.1101/2023.09.28.559962. bioRxiv. 2023. Update in: G3 (Bethesda). 2024 Apr 3;14(4):jkae030. doi: 10.1093/g3journal/jkae030 PMID: 38196611 Free PMC article. Updated. Preprint.
-
DAXX promotes centromeric stability independently of ATRX by preventing the accumulation of R-loop-induced DNA double-stranded breaks.Nucleic Acids Res. 2024 Feb 9;52(3):1136-1155. doi: 10.1093/nar/gkad1141. Nucleic Acids Res. 2024. PMID: 38038252 Free PMC article.
-
Proteasome Inhibition Reprograms Chromatin Landscape in Breast Cancer.Cancer Res Commun. 2024 Apr 16;4(4):1082-1099. doi: 10.1158/2767-9764.CRC-23-0476. Cancer Res Commun. 2024. PMID: 38625038 Free PMC article.
References
Publication types
MeSH terms
Substances
Grants and funding
LinkOut - more resources
Full Text Sources
Other Literature Sources
Miscellaneous