Single nuclei transcriptomics delineates complex immune and kidney cell interactions contributing to kidney allograft fibrosis
- PMID: 36863444
- PMCID: PMC10200746
- DOI: 10.1016/j.kint.2023.02.018
Single nuclei transcriptomics delineates complex immune and kidney cell interactions contributing to kidney allograft fibrosis
Abstract
Chronic allograft dysfunction (CAD), characterized histologically by interstitial fibrosis and tubular atrophy, is the major cause of kidney allograft loss. Here, using single nuclei RNA sequencing and transcriptome analysis, we identified the origin, functional heterogeneity, and regulation of fibrosis-forming cells in kidney allografts with CAD. A robust technique was used to isolate individual nuclei from kidney allograft biopsies and successfully profiled 23,980 nuclei from five kidney transplant recipients with CAD and 17,913 nuclei from three patients with normal allograft function. Our analysis revealed two distinct states of fibrosis in CAD; low and high extracellular matrix (ECM) with distinct kidney cell subclusters, immune cell types, and transcriptional profiles. Imaging mass cytometry analysis confirmed increased ECM deposition at the protein level. Proximal tubular cells transitioned to an injured mixed tubular (MT1) phenotype comprised of activated fibroblasts and myofibroblast markers, generated provisional ECM which recruited inflammatory cells, and served as the main driver of fibrosis. MT1 cells in the high ECM state achieved replicative repair evidenced by dedifferentiation and nephrogenic transcriptional signatures. MT1 in the low ECM state showed decreased apoptosis, decreased cycling tubular cells, and severe metabolic dysfunction, limiting the potential for repair. Activated B, T and plasma cells were increased in the high ECM state, while macrophage subtypes were increased in the low ECM state. Intercellular communication between kidney parenchymal cells and donor-derived macrophages, detected several years post-transplantation, played a key role in injury propagation. Thus, our study identified novel molecular targets for interventions aimed to ameliorate or prevent allograft fibrogenesis in kidney transplant recipients.
Keywords: chronic kidney injury; fibrosis development; kidney transplantation; snRNA-seq.
Copyright © 2023 International Society of Nephrology. Published by Elsevier Inc. All rights reserved.
Figures
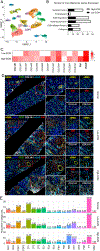
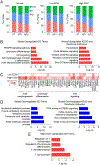
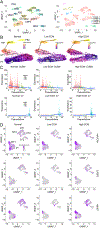
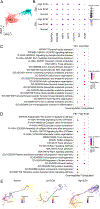
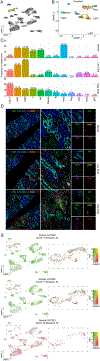
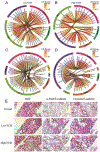
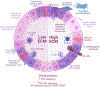
Similar articles
-
Detection of infiltrating fibroblasts by single-cell transcriptomics in human kidney allografts.PLoS One. 2022 Jun 3;17(6):e0267704. doi: 10.1371/journal.pone.0267704. eCollection 2022. PLoS One. 2022. PMID: 35657798 Free PMC article.
-
Renin-Angiotensin System Blockage and Avoiding High Doses of Calcineurin Inhibitors Prevent Interstitial Fibrosis and Tubular Atrophy in Kidney Transplant Recipients.Exp Clin Transplant. 2017 Feb;15(Suppl 1):32-36. doi: 10.6002/ect.mesot2016.O19. Exp Clin Transplant. 2017. PMID: 28260428
-
Antagonism of profibrotic microRNA-21 improves outcome of murine chronic renal allograft dysfunction.Kidney Int. 2017 Sep;92(3):646-656. doi: 10.1016/j.kint.2017.02.012. Epub 2017 Apr 8. Kidney Int. 2017. PMID: 28396121
-
Pathogenesis of Chronic Allograft Dysfunction Progress to Renal Fibrosis.Adv Exp Med Biol. 2019;1165:101-116. doi: 10.1007/978-981-13-8871-2_6. Adv Exp Med Biol. 2019. PMID: 31399963 Review.
-
Pathogenesis of tubulointerstitial fibrosis in chronic allograft dysfunction.Clin Transplant. 2009 Dec;23 Suppl 21:26-32. doi: 10.1111/j.1399-0012.2009.01106.x. Clin Transplant. 2009. PMID: 19930313 Review.
Cited by
-
The advance of single cell transcriptome to study kidney immune cells in diabetic kidney disease.BMC Nephrol. 2024 Nov 16;25(1):412. doi: 10.1186/s12882-024-03853-y. BMC Nephrol. 2024. PMID: 39550562 Free PMC article. Review.
-
Epithelial cell states associated with kidney and allograft injury.Nat Rev Nephrol. 2024 Jul;20(7):447-459. doi: 10.1038/s41581-024-00834-0. Epub 2024 Apr 17. Nat Rev Nephrol. 2024. PMID: 38632381 Free PMC article. Review.
-
Rictor/mTORC2 signalling contributes to renal vascular endothelial-to-mesenchymal transition and renal allograft interstitial fibrosis by regulating BNIP3-mediated mitophagy.Clin Transl Med. 2024 May;14(5):e1686. doi: 10.1002/ctm2.1686. Clin Transl Med. 2024. PMID: 38769658 Free PMC article.
-
Characterizing hub biomarkers for post-transplant renal fibrosis and unveiling their immunological functions through RNA sequencing and advanced machine learning techniques.J Transl Med. 2024 Feb 20;22(1):186. doi: 10.1186/s12967-024-04971-9. J Transl Med. 2024. PMID: 38378674 Free PMC article.
-
Predictive Model for Post-Transplant Renal Fibrosis Using Ultrasound Shear Wave Elastography.Ann Transplant. 2024 Oct 29;29:e945699. doi: 10.12659/AOT.945699. Ann Transplant. 2024. PMID: 39468406 Free PMC article.
References
Publication types
MeSH terms
Grants and funding
LinkOut - more resources
Full Text Sources
Medical
Miscellaneous