Mechanosensitive mTORC2 independently coordinates leading and trailing edge polarity programs during neutrophil migration
- PMID: 36857159
- PMCID: PMC10162419
- DOI: 10.1091/mbc.E22-05-0191
Mechanosensitive mTORC2 independently coordinates leading and trailing edge polarity programs during neutrophil migration
Abstract
By acting both upstream of and downstream from biochemical organizers of the cytoskeleton, physical forces function as central integrators of cell shape and movement. Here we use a combination of genetic, pharmacological, and optogenetic perturbations to probe the role of the conserved mechanosensitive mTOR complex 2 (mTORC2) programs in neutrophil polarity and motility. We find that the tension-based inhibition of leading-edge signals (Rac, F-actin) that underlies protrusion competition is gated by the kinase-independent role of the complex, whereas the regulation of RhoA and myosin II-based contractility at the trailing edge depend on mTORC2 kinase activity. mTORC2 is essential for spatial and temporal coordination of the front and back polarity programs for persistent migration under confinement. This mechanosensory pathway integrates multiple upstream signals, and we find that membrane stretch synergizes with biochemical co-input phosphatidylinositol (3,4,5)-trisphosphate to robustly amplify mTORC2 activation. Our results suggest that different signaling arms of mTORC2 regulate spatially and molecularly divergent cytoskeletal programs for efficient coordination of neutrophil shape and movement.
Figures
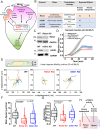
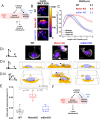
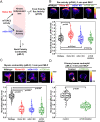
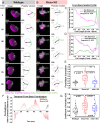
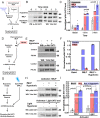
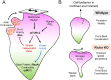
Similar articles
-
Membrane Tension Acts Through PLD2 and mTORC2 to Limit Actin Network Assembly During Neutrophil Migration.PLoS Biol. 2016 Jun 9;14(6):e1002474. doi: 10.1371/journal.pbio.1002474. eCollection 2016 Jun. PLoS Biol. 2016. PMID: 27280401 Free PMC article.
-
Mammalian target of rapamycin and Rictor control neutrophil chemotaxis by regulating Rac/Cdc42 activity and the actin cytoskeleton.Mol Biol Cell. 2013 Nov;24(21):3369-80. doi: 10.1091/mbc.E13-07-0405. Epub 2013 Sep 4. Mol Biol Cell. 2013. PMID: 24006489 Free PMC article.
-
mTORC1 and mTORC2 regulate EMT, motility, and metastasis of colorectal cancer via RhoA and Rac1 signaling pathways.Cancer Res. 2011 May 1;71(9):3246-56. doi: 10.1158/0008-5472.CAN-10-4058. Epub 2011 Mar 23. Cancer Res. 2011. PMID: 21430067 Free PMC article.
-
Ras, PI3K and mTORC2 - three's a crowd?J Cell Sci. 2020 Oct 8;133(19):jcs234930. doi: 10.1242/jcs.234930. J Cell Sci. 2020. PMID: 33033115 Free PMC article. Review.
-
Diverse signaling mechanisms of mTOR complexes: mTORC1 and mTORC2 in forming a formidable relationship.Adv Biol Regul. 2019 May;72:51-62. doi: 10.1016/j.jbior.2019.03.003. Epub 2019 Apr 11. Adv Biol Regul. 2019. PMID: 31010692 Review.
Cited by
-
Anoctamin-1 is induced by TGF-beta and contributes to lung myofibroblast differentiation.bioRxiv [Preprint]. 2023 Nov 9:2023.06.07.544093. doi: 10.1101/2023.06.07.544093. bioRxiv. 2023. Update in: Am J Physiol Lung Cell Mol Physiol. 2024 Jan 1;326(1):L111-L123. doi: 10.1152/ajplung.00155.2023 PMID: 37333255 Free PMC article. Updated. Preprint.
-
Excitable Rho dynamics control cell shape and motility by sequentially activating ERM proteins and actomyosin contractility.Sci Adv. 2024 Sep 6;10(36):eadn6858. doi: 10.1126/sciadv.adn6858. Epub 2024 Sep 6. Sci Adv. 2024. PMID: 39241071 Free PMC article.
-
Rictor-A Mediator of Progression and Metastasis in Lung Cancer.Cancers (Basel). 2024 Jan 26;16(3):543. doi: 10.3390/cancers16030543. Cancers (Basel). 2024. PMID: 38339294 Free PMC article. Review.
-
CRISPR-mediated reversion of oncogenic KRAS mutation results in increased proliferation and reveals independent roles of Ras and mTORC2 in the migration of A549 lung cancer cells.Mol Biol Cell. 2023 Dec 1;34(13):ar128. doi: 10.1091/mbc.E23-05-0152. Epub 2023 Sep 20. Mol Biol Cell. 2023. PMID: 37729017 Free PMC article.
-
MiR-155 enhances phagocytosis of alveolar macrophages through the mTORC2/RhoA pathway.Medicine (Baltimore). 2023 Sep 1;102(35):e34592. doi: 10.1097/MD.0000000000034592. Medicine (Baltimore). 2023. PMID: 37657048 Free PMC article.
References
-
- Bell GRR, Natwick DE, Collins SR (2018). Parallel High-Resolution Imaging of Leukocyte Chemotaxis Under Agarose with Rho-Family GTPase Biosensors. New York, NY: Humana Press, 71–85. - PubMed
-
- Berchtold D, Piccolis M, Chiaruttini N, Riezman I, Riezman H, Roux A, Walther TC, Loewith R (2012). Plasma membrane stress induces relocalization of Slm proteins and activation of TORC2 to promote sphingolipid synthesis. Nat Cell Biol 14, 542–547. - PubMed
Publication types
MeSH terms
Substances
Grants and funding
LinkOut - more resources
Full Text Sources
Research Materials
Miscellaneous