Senescent cancer cell-derived nanovesicle as a personalized therapeutic cancer vaccine
- PMID: 36854774
- PMCID: PMC10073290
- DOI: 10.1038/s12276-023-00951-z
Senescent cancer cell-derived nanovesicle as a personalized therapeutic cancer vaccine
Abstract
The development of therapeutic cancer vaccines (TCVs) that provide clinical benefits is challenging mainly due to difficulties in identifying immunogenic tumor antigens and effectively inducing antitumor immunity. Furthermore, there is an urgent need for personalized TCVs because only a limited number of tumor antigens are shared among cancer patients. Several autologous nanovaccines that do not require the identification of immunogenic tumor antigens have been proposed as personalized TCVs. However, these nanovaccines generally require exogenous adjuvants (e.g., Toll-like receptor agonists) to improve vaccine immunogenicity, which raises safety concerns. Here, we present senescent cancer cell-derived nanovesicle (SCCNV) as a personalized TCV that provides patient-specific tumor antigens and improved vaccine immunogenicity without the use of exogenous adjuvants. SCCNVs are prepared by inducing senescence in cancer cells ex vivo and subsequently extruding the senescent cancer cells through nanoporous membranes. In the clinical setting, SCCNVs can be prepared from autologous cancer cells from the blood of liquid tumor patients or from tumors surgically removed from solid cancer patients. SCCNVs also contain interferon-γ and tumor necrosis factor-α, which are expressed during senescence. These endogenous cytokines act as adjuvants and enhance vaccine immunogenicity, avoiding the need for exogenous adjuvants. Intradermally injected SCCNVs effectively activate dendritic cells and tumor-specific T cells and inhibit primary and metastatic tumor growth and tumor recurrence. SCCNV therapy showed an efficacy similar to that of immune checkpoint blockade (ICB) therapy and synergized with ICB. SCCNVs, which can be prepared using a simple and facile procedure, show potential as personalized TCVs.
© 2023. The Author(s).
Conflict of interest statement
The authors declare no competing interests.
Figures
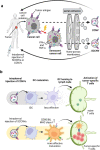
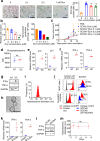
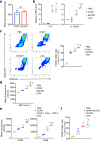
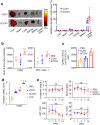
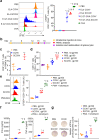
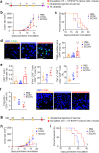
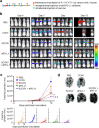
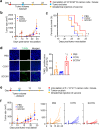
Similar articles
-
Monophosphoryl lipid A-assembled nanovaccines enhance tumor immunotherapy.Acta Biomater. 2023 Nov;171:482-494. doi: 10.1016/j.actbio.2023.09.013. Epub 2023 Sep 13. Acta Biomater. 2023. PMID: 37708924
-
Rational Design of T-Cell- and B-Cell-Based Therapeutic Cancer Vaccines.Acc Chem Res. 2022 Sep 20;55(18):2660-2671. doi: 10.1021/acs.accounts.2c00360. Epub 2022 Sep 1. Acc Chem Res. 2022. PMID: 36048514
-
Monophosphoryl lipid A (MPL) as an adjuvant for anti-cancer vaccines: clinical results.Adv Exp Med Biol. 2010;667:111-23. doi: 10.1007/978-1-4419-1603-7_10. Adv Exp Med Biol. 2010. PMID: 20665204
-
Vaccine-like nanomedicine for cancer immunotherapy.J Control Release. 2023 Mar;355:760-778. doi: 10.1016/j.jconrel.2023.02.015. Epub 2023 Feb 24. J Control Release. 2023. PMID: 36822241 Review.
-
Idiotypic vaccination for B-cell malignancies as a model for therapeutic cancer vaccines: from prototype protein to second generation vaccines.Haematologica. 2002 Sep;87(9):989-1001. Haematologica. 2002. PMID: 12217812 Review.
Cited by
-
Therapy-induced senescence through the redox lens.Redox Biol. 2024 Aug;74:103228. doi: 10.1016/j.redox.2024.103228. Epub 2024 Jun 6. Redox Biol. 2024. PMID: 38865902 Free PMC article. Review.
-
T-cell immunity against senescence: potential role and perspectives.Front Immunol. 2024 Mar 5;15:1360109. doi: 10.3389/fimmu.2024.1360109. eCollection 2024. Front Immunol. 2024. PMID: 38504990 Free PMC article. Review.
-
Engineering of Cell Derived-Nanovesicle as an Alternative to Exosome Therapy.Tissue Eng Regen Med. 2024 Jan;21(1):1-19. doi: 10.1007/s13770-023-00610-4. Epub 2023 Dec 8. Tissue Eng Regen Med. 2024. PMID: 38066355 Free PMC article. Review.
-
Nanoparticle-Based Immunotherapy for Reversing T-Cell Exhaustion.Int J Mol Sci. 2024 Jan 23;25(3):1396. doi: 10.3390/ijms25031396. Int J Mol Sci. 2024. PMID: 38338674 Free PMC article. Review.
-
Engineering Cell-Derived Nanovesicles for Targeted Immunomodulation.Nanomaterials (Basel). 2023 Oct 12;13(20):2751. doi: 10.3390/nano13202751. Nanomaterials (Basel). 2023. PMID: 37887902 Free PMC article.
References
Publication types
MeSH terms
Substances
LinkOut - more resources
Full Text Sources