The Actin Network Interfacing Diverse Integrin-Mediated Adhesions
- PMID: 36830665
- PMCID: PMC9953007
- DOI: 10.3390/biom13020294
The Actin Network Interfacing Diverse Integrin-Mediated Adhesions
Abstract
The interface between the cellular actin network and diverse forms of integrin-mediated cell adhesions displays a unique capacity to serve as accurate chemical and mechanical sensors of the cell's microenvironment. Focal adhesion-like structures of diverse cell types, podosomes in osteoclasts, and invadopodia of invading cancer cells display distinct morphologies and apparent functions. Yet, all three share a similar composition and mode of coupling between a protrusive structure (the lamellipodium, the core actin bundle of the podosome, and the invadopodia protrusion, respectively), and a nearby adhesion site. Cytoskeletal or external forces, applied to the adhesion sites, trigger a cascade of unfolding and activation of key adhesome components (e.g., talin, vinculin, integrin), which in turn, trigger the assembly of adhesion sites and generation of adhesion-mediated signals that affect cell behavior and fate. The structural and molecular mechanisms underlying the dynamic crosstalk between the actin cytoskeleton and the adhesome network are discussed.
Keywords: actin; cell–matrix adhesions; focal adhesions; integrins; invadopodia; podosomes; vinculin.
Conflict of interest statement
All authors declare that there are no conflicts of interest associated with this article.
Figures
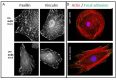
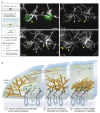
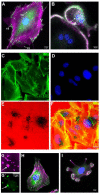
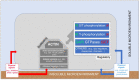
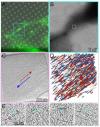
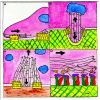
Similar articles
-
Early molecular events in the assembly of the focal adhesion-stress fiber complex during fibroblast spreading.Cell Motil Cytoskeleton. 2004 Jul;58(3):143-59. doi: 10.1002/cm.20005. Cell Motil Cytoskeleton. 2004. PMID: 15146534
-
Integrin-mediated cell adhesion: the cytoskeletal connection.Biochem Soc Symp. 1999;65:79-99. Biochem Soc Symp. 1999. PMID: 10320934 Review.
-
Biomechanical regulation of focal adhesion and invadopodia formation.J Cell Sci. 2020 Oct 22;133(20):jcs244848. doi: 10.1242/jcs.244848. J Cell Sci. 2020. PMID: 33093229 Review.
-
Talin-activated vinculin interacts with branched actin networks to initiate bundles.Elife. 2020 Nov 13;9:e53990. doi: 10.7554/eLife.53990. Elife. 2020. PMID: 33185186 Free PMC article.
-
Actin machinery and mechanosensitivity in invadopodia, podosomes and focal adhesions.J Cell Sci. 2009 Sep 1;122(Pt 17):3037-49. doi: 10.1242/jcs.052704. J Cell Sci. 2009. PMID: 19692590 Free PMC article. Review.
Cited by
-
A Regulator Role for the ATP-Binding Cassette Subfamily C Member 6 Transporter in HepG2 Cells: Effect on the Dynamics of Cell-Cell and Cell-Matrix Interactions.Int J Mol Sci. 2023 Nov 16;24(22):16391. doi: 10.3390/ijms242216391. Int J Mol Sci. 2023. PMID: 38003580 Free PMC article.
-
Actin Cytoskeleton and Integrin Components Are Interdependent for Slit Diaphragm Maintenance in Drosophila Nephrocytes.Cells. 2024 Aug 14;13(16):1350. doi: 10.3390/cells13161350. Cells. 2024. PMID: 39195240 Free PMC article.
-
Structure and function of the membrane microdomains in osteoclasts.Bone Res. 2023 Nov 21;11(1):61. doi: 10.1038/s41413-023-00294-5. Bone Res. 2023. PMID: 37989999 Free PMC article. Review.
-
The pseudoenzyme ADPRHL1 affects cardiac function by regulating the ROCK pathway.Stem Cell Res Ther. 2023 Oct 26;14(1):309. doi: 10.1186/s13287-023-03507-0. Stem Cell Res Ther. 2023. PMID: 37880701 Free PMC article.
References
Publication types
MeSH terms
Substances
Grants and funding
LinkOut - more resources
Full Text Sources