Comprehensive chromatin proteomics resolves functional phases of pluripotency and identifies changes in regulatory components
- PMID: 36806742
- PMCID: PMC10085704
- DOI: 10.1093/nar/gkad058
Comprehensive chromatin proteomics resolves functional phases of pluripotency and identifies changes in regulatory components
Abstract
The establishment of cellular identity is driven by transcriptional and epigenetic regulators of the chromatin proteome - the chromatome. Comprehensive analyses of the chromatome composition and dynamics can therefore greatly improve our understanding of gene regulatory mechanisms. Here, we developed an accurate mass spectrometry (MS)-based proteomic method called Chromatin Aggregation Capture (ChAC) followed by Data-Independent Acquisition (DIA) and analyzed chromatome reorganizations during major phases of pluripotency. This enabled us to generate a comprehensive atlas of proteomes, chromatomes, and chromatin affinities for the ground, formative and primed pluripotency states, and to pinpoint the specific binding and rearrangement of regulatory components. These comprehensive datasets combined with extensive analyses identified phase-specific factors like QSER1 and JADE1/2/3 and provide a detailed foundation for an in-depth understanding of mechanisms that govern the phased progression of pluripotency. The technical advances reported here can be readily applied to other models in development and disease.
© The Author(s) 2023. Published by Oxford University Press on behalf of Nucleic Acids Research.
Figures
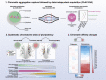
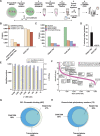
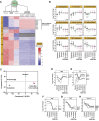
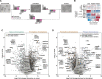
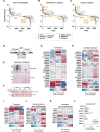
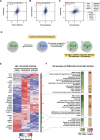
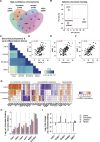
Similar articles
-
DNA and chromatin modification networks distinguish stem cell pluripotent ground states.Mol Cell Proteomics. 2012 Oct;11(10):1036-47. doi: 10.1074/mcp.M111.011114. Epub 2012 Jul 22. Mol Cell Proteomics. 2012. PMID: 22822199 Free PMC article.
-
Multi-omic Profiling Reveals Dynamics of the Phased Progression of Pluripotency.Cell Syst. 2019 May 22;8(5):427-445.e10. doi: 10.1016/j.cels.2019.03.012. Epub 2019 May 8. Cell Syst. 2019. PMID: 31078527 Free PMC article.
-
A Mass Spectrometry Survey of Chromatin-Associated Proteins in Pluripotency and Early Lineage Commitment.Proteomics. 2019 Jul;19(14):e1900047. doi: 10.1002/pmic.201900047. Epub 2019 Jul 3. Proteomics. 2019. PMID: 31219242
-
Proteotyping pluripotency with mass spectrometry.Expert Rev Proteomics. 2019 May;16(5):391-400. doi: 10.1080/14789450.2019.1604229. Epub 2019 Apr 14. Expert Rev Proteomics. 2019. PMID: 30947573 Review.
-
Proteomics and pluripotency.Crit Rev Biochem Mol Biol. 2011 Dec;46(6):493-506. doi: 10.3109/10409238.2011.624491. Epub 2011 Oct 15. Crit Rev Biochem Mol Biol. 2011. PMID: 21999516 Free PMC article. Review.
Cited by
-
Non-canonical functions of UHRF1 maintain DNA methylation homeostasis in cancer cells.Nat Commun. 2024 Apr 5;15(1):2960. doi: 10.1038/s41467-024-47314-4. Nat Commun. 2024. PMID: 38580649 Free PMC article.
-
DNA methylation shapes the Polycomb landscape during the exit from naive pluripotency.Nat Struct Mol Biol. 2024 Oct 24. doi: 10.1038/s41594-024-01405-4. Online ahead of print. Nat Struct Mol Biol. 2024. PMID: 39448850
References
Publication types
MeSH terms
Substances
LinkOut - more resources
Full Text Sources