QuilA® adjuvanted Coxevac® sustains Th1-CD8+-type immunity and increases protection in Coxiella burnetii-challenged goats
- PMID: 36788233
- PMCID: PMC9929268
- DOI: 10.1038/s41541-023-00607-z
QuilA® adjuvanted Coxevac® sustains Th1-CD8+-type immunity and increases protection in Coxiella burnetii-challenged goats
Abstract
Coxevac® is the EMA-approved veterinary vaccine for the protection of cattle and goats against Q fever, a zoonotic bacterial disease due to Coxiella burnetii. Since Coxevac® reduces bacterial shedding and clinical symptoms but does not prevent infection, novel, ready-to-use vaccine formulations are needed to increase its immunogenicity. Here, a goat vaccination-challenge model was used to evaluate the impact of the commercially available saponin-based QuilA® adjuvant on Coxevac® immunity. Upon challenge, the QuilA®-Coxevac® group showed a stronger immune response reflected in a higher magnitude of total IgG and an increase in circulating and splenic CD8+ T-cells compared to the Coxevac® and challenged-control groups. The QuilA®-Coxevac® group was characterized by a targeted Th1-type response (IFNγ, IP10) associated with increased transcripts of CD8+ and NK cells in spleens and γδ T cells in bronchial lymph nodes. Coxevac® vaccinated animals presented an intermediate expression of Th1-related genes, while the challenged-control group showed an immune response characterized by pro-inflammatory (IL1β, TNFα, IL12), Th2 (IL4 and IL13), Th17 (IL17A) and other immunoregulatory cytokines (IL6, IL10). An intriguing role was observed for γδ T cells, which were of TBX21- and SOX4-types in the QuilA®-Coxevac® and challenged control group, respectively. Overall, the addition of QuilA® resulted in a sustained Th1-type activation associated with an increased vaccine-induced bacterial clearance of 33.3% as compared to Coxevac® only. QuilA® could be proposed as a readily-applied veterinary solution to improve Coxevac® efficacy against C. burnetii infection in field settings.
© 2023. The Author(s).
Conflict of interest statement
The authors declare no competing interests.
Figures
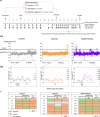
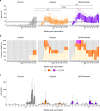
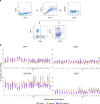
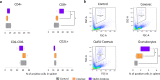
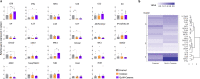
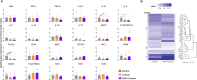
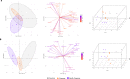
Similar articles
-
Efficacy of Phase I and Phase II Coxiella burnetii Bacterin Vaccines in a Pregnant Ewe Challenge Model.Vaccines (Basel). 2023 Feb 22;11(3):511. doi: 10.3390/vaccines11030511. Vaccines (Basel). 2023. PMID: 36992095 Free PMC article.
-
Humoral and cellular immune responses in sheep following administration of different doses of an inactivated phase I vaccine against Coxiella burnetii.Vaccine. 2023 Jul 25;41(33):4798-4807. doi: 10.1016/j.vaccine.2023.06.061. Epub 2023 Jun 23. Vaccine. 2023. PMID: 37357077
-
Effect of a phase I Coxiella burnetii inactivated vaccine on body temperature and milk yield in dairy cows.J Dairy Sci. 2016 Jan;99(1):541-50. doi: 10.3168/jds.2015-9628. Epub 2015 Nov 5. J Dairy Sci. 2016. PMID: 26547657
-
A systematic review and meta-analysis of phase I inactivated vaccines to reduce shedding of Coxiella burnetii from sheep and goats from routes of public health importance.Zoonoses Public Health. 2014 Dec;61(8):519-33. doi: 10.1111/zph.12086. Epub 2013 Nov 19. Zoonoses Public Health. 2014. PMID: 24251777 Review.
-
Coxiella burnetii infections in sheep or goats: an opinionated review.Vet Microbiol. 2015 Dec 14;181(1-2):119-29. doi: 10.1016/j.vetmic.2015.07.011. Epub 2015 Jul 15. Vet Microbiol. 2015. PMID: 26315774 Review.
Cited by
-
Efficacy and Safety of an Inactivated Phase I Coxiella burnetii Vaccine to Control Q Fever in Ruminants: A Systematic Review.Animals (Basel). 2024 May 16;14(10):1484. doi: 10.3390/ani14101484. Animals (Basel). 2024. PMID: 38791700 Free PMC article. Review.
-
Q fever immunology: the quest for a safe and effective vaccine.NPJ Vaccines. 2023 Sep 7;8(1):133. doi: 10.1038/s41541-023-00727-6. NPJ Vaccines. 2023. PMID: 37679410 Free PMC article. Review.
-
Synthetic Particulate Subunit Vaccines for the Prevention of Q Fever.Adv Healthc Mater. 2024 Mar;13(7):e2302351. doi: 10.1002/adhm.202302351. Epub 2024 Jan 16. Adv Healthc Mater. 2024. PMID: 38198823 Free PMC article.
References
LinkOut - more resources
Full Text Sources
Research Materials