Beyond Pellagra-Research Models and Strategies Addressing the Enduring Clinical Relevance of NAD Deficiency in Aging and Disease
- PMID: 36766842
- PMCID: PMC9913999
- DOI: 10.3390/cells12030500
Beyond Pellagra-Research Models and Strategies Addressing the Enduring Clinical Relevance of NAD Deficiency in Aging and Disease
Abstract
Research into the functions of nicotinamide adenine dinucleotide (NAD) has intensified in recent years due to the insight that abnormally low levels of NAD are involved in many human pathologies including metabolic disorders, neurodegeneration, reproductive dysfunction, cancer, and aging. Consequently, the development and validation of novel NAD-boosting strategies has been of central interest, along with the development of models that accurately represent the complexity of human NAD dynamics and deficiency levels. In this review, we discuss pioneering research and show how modern researchers have long since moved past believing that pellagra is the overt and most dramatic clinical presentation of NAD deficiency. The current research is centered on common human health conditions associated with moderate, but clinically relevant, NAD deficiency. In vitro and in vivo research models that have been developed specifically to study NAD deficiency are reviewed here, along with emerging strategies to increase the intracellular NAD concentrations.
Keywords: CD38; NAD; NAD animal models; aging; niacin; niacin deficiency; nicotinamide; nicotinic acid; poly(ADP-ribose); sirtuin.
Conflict of interest statement
The authors declare no conflict of interest. The funders had no role in the writing of the manuscript.
Figures
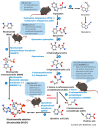
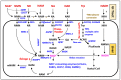
Similar articles
-
Niacin.Adv Food Nutr Res. 2018;83:83-149. doi: 10.1016/bs.afnr.2017.11.003. Epub 2018 Feb 1. Adv Food Nutr Res. 2018. PMID: 29477227
-
TNB-738, a biparatopic antibody, boosts intracellular NAD+ by inhibiting CD38 ecto-enzyme activity.MAbs. 2022 Jan-Dec;14(1):2095949. doi: 10.1080/19420862.2022.2095949. MAbs. 2022. PMID: 35867844 Free PMC article.
-
NAD+ and sirtuins in aging and disease.Trends Cell Biol. 2014 Aug;24(8):464-71. doi: 10.1016/j.tcb.2014.04.002. Epub 2014 Apr 29. Trends Cell Biol. 2014. PMID: 24786309 Free PMC article. Review.
-
Role of Nicotinamide Adenine Dinucleotide and Related Precursors as Therapeutic Targets for Age-Related Degenerative Diseases: Rationale, Biochemistry, Pharmacokinetics, and Outcomes.Antioxid Redox Signal. 2019 Jan 10;30(2):251-294. doi: 10.1089/ars.2017.7269. Epub 2018 May 11. Antioxid Redox Signal. 2019. PMID: 29634344 Free PMC article. Review.
-
COVID-19: Are We Facing Secondary Pellagra Which Cannot Simply Be Cured by Vitamin B3?Int J Mol Sci. 2022 Apr 13;23(8):4309. doi: 10.3390/ijms23084309. Int J Mol Sci. 2022. PMID: 35457123 Free PMC article. Review.
Cited by
-
A metabolic signature for NADSYN1-dependent congenital NAD deficiency disorder.J Clin Invest. 2024 Feb 15;134(4):e174824. doi: 10.1172/JCI174824. J Clin Invest. 2024. PMID: 38357931 Free PMC article.
-
Regulation of and challenges in targeting NAD+ metabolism.Nat Rev Mol Cell Biol. 2024 Oct;25(10):822-840. doi: 10.1038/s41580-024-00752-w. Epub 2024 Jul 18. Nat Rev Mol Cell Biol. 2024. PMID: 39026037 Review.
-
NAD+ Precursors and Intestinal Inflammation: Therapeutic Insights Involving Gut Microbiota.Nutrients. 2023 Jun 30;15(13):2992. doi: 10.3390/nu15132992. Nutrients. 2023. PMID: 37447318 Free PMC article. Review.
-
Differential responses to maternal diabetes in embryo and visceral yolk sac.Front Cell Dev Biol. 2023 Oct 19;11:1273641. doi: 10.3389/fcell.2023.1273641. eCollection 2023. Front Cell Dev Biol. 2023. PMID: 37928898 Free PMC article.
-
The role of nicotinamide adenine dinucleotide salvage enzymes in cardioprotection.Kardiochir Torakochirurgia Pol. 2024 Jun;21(2):86-95. doi: 10.5114/kitp.2024.141145. Epub 2024 Jun 30. Kardiochir Torakochirurgia Pol. 2024. PMID: 39055245 Free PMC article. Review.
References
-
- Harden A., Young W.J. The Alcoholic Ferment of Yeast-Juice. Proc. R. Soc. Lond. B. 1906;77:405–420. doi: 10.1098/rspb.1906.0029. - DOI
-
- Warburg O., Christian J.W.B.Z. Pyridin, the Hydrogen-Transferring Component of the Fermentation Enzymes (Pyridine Nucleotide) Biochem. Z. 1936;287:1.
-
- Chambon P., Weill J.D., Doly J., Strosser M.T., Mandel P. On the Formation of a Novel Adenylic Compound by Enzymatic Extracts of Liver Nuclei. Biochem. Biophys. Res. Commun. 1966;25:638–643. doi: 10.1016/0006-291X(66)90502-X. - DOI
Publication types
MeSH terms
Substances
Grants and funding
LinkOut - more resources
Full Text Sources
Research Materials
Miscellaneous