Structural insights into catalytical capability for CPT11 hydrolysis and substrate specificity of a novel marine microbial carboxylesterase, E93
- PMID: 36756200
- PMCID: PMC9901791
- DOI: 10.3389/fmicb.2022.1081094
Structural insights into catalytical capability for CPT11 hydrolysis and substrate specificity of a novel marine microbial carboxylesterase, E93
Abstract
Introduction: CPT11 (Irinotecan; 7-ethyl-10-[4-(1-piperidino)-1-piperidino] carbonyloxycamptothecin) is an important camptothecin-based broad-spectrum anticancer prodrug. The activation of its warhead, SN38 (7-ethyl-10-hydroxycamptothecin), requires hydrolysis by carboxylesterases. NPC (7-ethyl-10-[4-(1-piperidino)-1-amino] carbonyloxycamptothecin) is a metabolic derivative of CPT11 and is difficult to be hydrolyzed by human carboxylesterase. Microbial carboxylesterase with capability on both CPT11 and NPC hydrolysis is rarely reported. A marine microbial carboxylesterase, E93, was identified to hydrolyze both substrates in this study. This enzyme was an appropriate subject for uncovering the catalytic mechanism of carboxylesterases to CPT11 and NPC hydrolysis.
Methods: X-ray diffraction method was applied to obtain high-resolution structure of E93. Molecular docking was adopted to analyze the interaction of E93 with p-NP (p-nitrophenyl), CPT11, and NPC substrates. Mutagenesis and enzymatic assay were adopted to verify the binding pattern of substrates.
Results: Three core regions (Region A, B, and C) of the catalytic pocket were identified and their functions on substrates specificity were validated via mutagenesis assays. The Region A was involved in the binding with the alcohol group of all tested substrates. The size and hydrophobicity of the region determined the binding affinity. The Region B accommodated the acyl group of p-NP and CPT11 substrates. The polarity of this region determined the catalytic preference to both substrates. The Region C specifically accommodated the acyl group of NPC. The interaction from the acidic residue, E428, contributed to the binding of E93 with NPC.
Discussion: The study analyzed both unique and conserved structures of the pocket in E93, for the first time demonstrating the discrepancy of substrate-enzyme interaction between CPT11 and NPC. It also expanded the knowledge about the substrate specificity and potential application of microbial Family VII carboxylesterases.
Keywords: crystal structure; enzyme catalysis; marine bacterial carboxylesterase; p-nitrophenyl; prodrug; substrate specificity.
Copyright © 2023 Li, Rong, Li, Cui, Li and Xu.
Conflict of interest statement
The authors declare that the research was conducted in the absence of any commercial or financial relationships that could be construed as a potential conflict of interest.
Figures
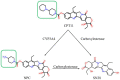
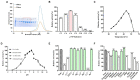
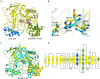
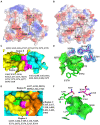
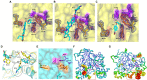
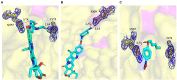
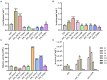
Similar articles
-
Hydrolysis of irinotecan and its oxidative metabolites, 7-ethyl-10-[4-N-(5-aminopentanoic acid)-1-piperidino] carbonyloxycamptothecin and 7-ethyl-10-[4-(1-piperidino)-1-amino]-carbonyloxycamptothecin, by human carboxylesterases CES1A1, CES2, and a newly expressed carboxylesterase isoenzyme, CES3.Drug Metab Dispos. 2004 May;32(5):505-11. doi: 10.1124/dmd.32.5.505. Drug Metab Dispos. 2004. PMID: 15100172
-
Carboxylesterase isoform 2 mRNA expression in peripheral blood mononuclear cells is a predictive marker of the irinotecan to SN38 activation step in colorectal cancer patients.Clin Cancer Res. 2005 Oct 1;11(19 Pt 1):6901-7. doi: 10.1158/1078-0432.CCR-05-0602. Clin Cancer Res. 2005. PMID: 16203781
-
Mouse liver and kidney carboxylesterase (M-LK) rapidly hydrolyzes antitumor prodrug irinotecan and the N-terminal three quarter sequence determines substrate selectivity.Drug Metab Dispos. 2003 Jan;31(1):21-7. doi: 10.1124/dmd.31.1.21. Drug Metab Dispos. 2003. PMID: 12485949
-
Applications of carboxylesterase activity in environmental monitoring and toxicity identification evaluations (TIEs).Rev Environ Contam Toxicol. 2008;195:117-78. doi: 10.1007/978-0-387-77030-7_5. Rev Environ Contam Toxicol. 2008. PMID: 18418956 Review.
-
Microbiota-Host-Irinotecan Axis: A New Insight Toward Irinotecan Chemotherapy.Front Cell Infect Microbiol. 2021 Oct 14;11:710945. doi: 10.3389/fcimb.2021.710945. eCollection 2021. Front Cell Infect Microbiol. 2021. PMID: 34722328 Free PMC article. Review.
Cited by
-
Identification and expression of MarCE, a marine carboxylesterase with synthetic ester-degrading activity.Microb Biotechnol. 2024 Jun;17(6):e14479. doi: 10.1111/1751-7915.14479. Microb Biotechnol. 2024. PMID: 38881500 Free PMC article.
References
LinkOut - more resources
Full Text Sources
Miscellaneous