This is a preprint.
The mitochondrial multi-omic response to exercise training across tissues
- PMID: 36711881
- PMCID: PMC9882193
- DOI: 10.1101/2023.01.13.523698
The mitochondrial multi-omic response to exercise training across tissues
Update in
-
The mitochondrial multi-omic response to exercise training across rat tissues.Cell Metab. 2024 Jun 4;36(6):1411-1429.e10. doi: 10.1016/j.cmet.2023.12.021. Epub 2024 May 2. Cell Metab. 2024. PMID: 38701776
Abstract
Mitochondria are adaptable organelles with diverse cellular functions critical to whole-body metabolic homeostasis. While chronic endurance exercise training is known to alter mitochondrial activity, these adaptations have not yet been systematically characterized. Here, the Molecular Transducers of Physical Activity Consortium (MoTrPAC) mapped the longitudinal, multi-omic changes in mitochondrial analytes across 19 tissues in male and female rats endurance trained for 1, 2, 4 or 8 weeks. Training elicited substantial changes in the adrenal gland, brown adipose, colon, heart and skeletal muscle, while we detected mild responses in the brain, lung, small intestine and testes. The colon response was characterized by non-linear dynamics that resulted in upregulation of mitochondrial function that was more prominent in females. Brown adipose and adrenal tissues were characterized by substantial downregulation of mitochondrial pathways. Training induced a previously unrecognized robust upregulation of mitochondrial protein abundance and acetylation in the liver, and a concomitant shift in lipid metabolism. The striated muscles demonstrated a highly coordinated response to increase oxidative capacity, with the majority of changes occurring in protein abundance and post-translational modifications. We identified exercise upregulated networks that are downregulated in human type 2 diabetes and liver cirrhosis. In both cases HSD17B10, a central dehydrogenase in multiple metabolic pathways and mitochondrial tRNA maturation, was the main hub. In summary, we provide a multi-omic, cross-tissue atlas of the mitochondrial response to training and identify candidates for prevention of disease-associated mitochondrial dysfunction.
Figures
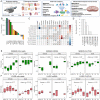
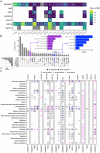
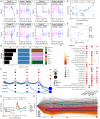
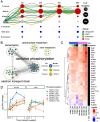
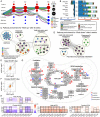
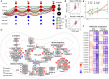
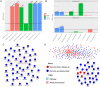
Similar articles
-
The mitochondrial multi-omic response to exercise training across rat tissues.Cell Metab. 2024 Jun 4;36(6):1411-1429.e10. doi: 10.1016/j.cmet.2023.12.021. Epub 2024 May 2. Cell Metab. 2024. PMID: 38701776
-
Sexual dimorphism and the multi-omic response to exercise training in rat subcutaneous white adipose tissue.bioRxiv [Preprint]. 2023 Feb 4:2023.02.03.527012. doi: 10.1101/2023.02.03.527012. bioRxiv. 2023. Update in: Nat Metab. 2024 May;6(5):963-979. doi: 10.1038/s42255-023-00959-9. PMID: 36778330 Free PMC article. Updated. Preprint.
-
Molecular Transducers of Physical Activity Consortium (MoTrPAC): human studies design and protocol.J Appl Physiol (1985). 2024 Sep 1;137(3):473-493. doi: 10.1152/japplphysiol.00102.2024. Epub 2024 Apr 18. J Appl Physiol (1985). 2024. PMID: 38634503
-
Exercise Effects on White Adipose Tissue: Beiging and Metabolic Adaptations.Diabetes. 2015 Jul;64(7):2361-8. doi: 10.2337/db15-0227. Epub 2015 Jun 7. Diabetes. 2015. PMID: 26050668 Free PMC article. Review.
-
Molecular Transducers of Physical Activity Consortium (MoTrPAC): Mapping the Dynamic Responses to Exercise.Cell. 2020 Jun 25;181(7):1464-1474. doi: 10.1016/j.cell.2020.06.004. Cell. 2020. PMID: 32589957 Free PMC article. Review.
References
-
- Kluck R. M., Bossy-Wetzel E., Green D. R. & Newmeyer D. D. The release of cytochrome c from mitochondria: a primary site for Bcl-2 regulation of apoptosis. Science 275, 1132–1136 (1997). - PubMed
-
- Mootha V. K. et al. Integrated analysis of protein composition, tissue diversity, and gene regulation in mouse mitochondria. Cell 115, 629–640 (2003). - PubMed
-
- Kappler L. et al. Linking bioenergetic function of mitochondria to tissue-specific molecular fingerprints. Am. J. Physiol. Endocrinol. Metab. 317, E374–E387 (2019). - PubMed
Publication types
Grants and funding
- U01 AG055135/AG/NIA NIH HHS/United States
- U01 AR071158/AR/NIAMS NIH HHS/United States
- U01 AG055137/AG/NIA NIH HHS/United States
- U01 AR071130/AR/NIAMS NIH HHS/United States
- U01 AR071133/AR/NIAMS NIH HHS/United States
- U24 DK112340/DK/NIDDK NIH HHS/United States
- U24 DK112341/DK/NIDDK NIH HHS/United States
- P30 DK020541/DK/NIDDK NIH HHS/United States
- U24 DK112326/DK/NIDDK NIH HHS/United States
- U24 OD026629/OD/NIH HHS/United States
- U24 DK112342/DK/NIDDK NIH HHS/United States
- U24 DK112331/DK/NIDDK NIH HHS/United States
- U24 AR071113/AR/NIAMS NIH HHS/United States
- U01 AR071160/AR/NIAMS NIH HHS/United States
- U24 DK112348/DK/NIDDK NIH HHS/United States
- U01 AG055133/AG/NIA NIH HHS/United States
- U01 AR071128/AR/NIAMS NIH HHS/United States
- U01 AR071124/AR/NIAMS NIH HHS/United States
- U01 AG070928/AG/NIA NIH HHS/United States
- U24 DK112349/DK/NIDDK NIH HHS/United States
- U01 AR071150/AR/NIAMS NIH HHS/United States
LinkOut - more resources
Full Text Sources
Miscellaneous