The enterovirus genome can be translated in an IRES-independent manner that requires the initiation factors eIF2A/eIF2D
- PMID: 36689548
- PMCID: PMC9894558
- DOI: 10.1371/journal.pbio.3001693
The enterovirus genome can be translated in an IRES-independent manner that requires the initiation factors eIF2A/eIF2D
Abstract
RNA recombination in positive-strand RNA viruses is a molecular-genetic process, which permits the greatest evolution of the genome and may be essential to stabilizing the genome from the deleterious consequences of accumulated mutations. Enteroviruses represent a useful system to elucidate the details of this process. On the biochemical level, it is known that RNA recombination is catalyzed by the viral RNA-dependent RNA polymerase using a template-switching mechanism. For this mechanism to function in cells, the recombining genomes must be located in the same subcellular compartment. How a viral genome is trafficked to the site of genome replication and recombination, which is membrane associated and isolated from the cytoplasm, is not known. We hypothesized that genome translation was essential for colocalization of genomes for recombination. We show that complete inactivation of internal ribosome entry site (IRES)-mediated translation of a donor enteroviral genome enhanced recombination instead of impairing it. Recombination did not occur by a nonreplicative mechanism. Rather, sufficient translation of the nonstructural region of the genome occurred to support subsequent steps required for recombination. The noncanonical translation initiation factors, eIF2A and eIF2D, were required for IRES-independent translation. Our results support an eIF2A/eIF2D-dependent mechanism under conditions in which the eIF2-dependent mechanism is inactive. Detection of an IRES-independent mechanism for translation of the enterovirus genome provides an explanation for a variety of debated observations, including nonreplicative recombination and persistence of enteroviral RNA lacking an IRES. The existence of an eIF2A/eIF2D-dependent mechanism in enteroviruses predicts the existence of similar mechanisms in other viruses.
Copyright: © 2023 Kim et al. This is an open access article distributed under the terms of the Creative Commons Attribution License, which permits unrestricted use, distribution, and reproduction in any medium, provided the original author and source are credited.
Conflict of interest statement
The authors have declared that no competing interests exist.
Figures
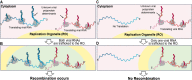
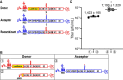

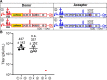
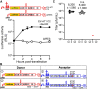
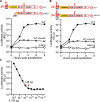
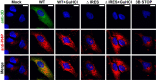
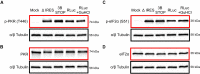
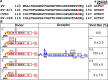
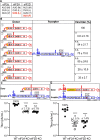
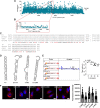
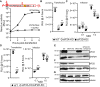
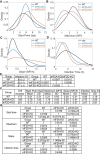
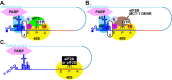
Comment in
-
Existence and significance of viral nonreplicative RNA recombination.PLoS Biol. 2023 Jul 25;21(7):e3002216. doi: 10.1371/journal.pbio.3002216. eCollection 2023 Jul. PLoS Biol. 2023. PMID: 37490454 Free PMC article.
Similar articles
-
Hepatitis C Virus Translation Regulation.Int J Mol Sci. 2020 Mar 27;21(7):2328. doi: 10.3390/ijms21072328. Int J Mol Sci. 2020. PMID: 32230899 Free PMC article. Review.
-
The Initiation Factors eIF2, eIF2A, eIF2D, eIF4A, and eIF4G Are Not Involved in Translation Driven by Hepatitis C Virus IRES in Human Cells.Front Microbiol. 2018 Feb 13;9:207. doi: 10.3389/fmicb.2018.00207. eCollection 2018. Front Microbiol. 2018. PMID: 29487587 Free PMC article.
-
Translation of Sindbis Subgenomic mRNA is Independent of eIF2, eIF2A and eIF2D.Sci Rep. 2017 Feb 27;7:43876. doi: 10.1038/srep43876. Sci Rep. 2017. PMID: 28240315 Free PMC article.
-
Nuclear Protein Sam68 Interacts with the Enterovirus 71 Internal Ribosome Entry Site and Positively Regulates Viral Protein Translation.J Virol. 2015 Oct;89(19):10031-43. doi: 10.1128/JVI.01677-15. Epub 2015 Jul 22. J Virol. 2015. PMID: 26202240 Free PMC article.
-
Advances and Breakthroughs in IRES-Directed Translation and Replication of Picornaviruses.mBio. 2023 Apr 25;14(2):e0035823. doi: 10.1128/mbio.00358-23. Epub 2023 Mar 20. mBio. 2023. PMID: 36939331 Free PMC article. Review.
Cited by
-
Neoantigen vaccine nanoformulations based on Chemically synthesized minimal mRNA (CmRNA): small molecules, big impact.NPJ Vaccines. 2024 Jan 18;9(1):14. doi: 10.1038/s41541-024-00807-1. NPJ Vaccines. 2024. PMID: 38238340 Free PMC article. Review.
-
Candidate genes for field resistance to cassava brown streak disease revealed through the analysis of multiple data sources.Front Plant Sci. 2023 Nov 3;14:1270963. doi: 10.3389/fpls.2023.1270963. eCollection 2023. Front Plant Sci. 2023. PMID: 38023930 Free PMC article.
-
Hepatoviruses promote very-long-chain fatty acid and sphingolipid synthesis for viral RNA replication and quasi-enveloped virus release.Sci Adv. 2023 Oct 20;9(42):eadj4198. doi: 10.1126/sciadv.adj4198. Epub 2023 Oct 20. Sci Adv. 2023. PMID: 37862421 Free PMC article.
-
Existence and significance of viral nonreplicative RNA recombination.PLoS Biol. 2023 Jul 25;21(7):e3002216. doi: 10.1371/journal.pbio.3002216. eCollection 2023 Jul. PLoS Biol. 2023. PMID: 37490454 Free PMC article.
-
Structure and function of type IV IRES in picornaviruses: a systematic review.Front Microbiol. 2024 May 24;15:1415698. doi: 10.3389/fmicb.2024.1415698. eCollection 2024. Front Microbiol. 2024. PMID: 38855772 Free PMC article.
References
Publication types
MeSH terms
Substances
Grants and funding
LinkOut - more resources
Full Text Sources
Research Materials