TGF-β/IFN-γ Antagonism in Subversion and Self-Defense of Phase II Coxiella burnetii - Infected Dendritic Cells
- PMID: 36688662
- PMCID: PMC9933720
- DOI: 10.1128/iai.00323-22
TGF-β/IFN-γ Antagonism in Subversion and Self-Defense of Phase II Coxiella burnetii - Infected Dendritic Cells
Abstract
Dendritic cells (DCs) belong to the first line of innate defense and come into early contact with invading pathogens, including the zoonotic bacterium Coxiella burnetii, the causative agent of Q fever. However, the pathogen-host cell interactions in C. burnetii-infected DCs, particularly the role of mechanisms of immune subversion beyond virulent phase I lipopolysaccharide (LPS), as well as the contribution of cellular self-defense strategies, are not understood. Using phase II Coxiella-infected DCs, we show that impairment of DC maturation and MHC I downregulation is caused by autocrine release and action of immunosuppressive transforming growth factor-β (TGF-β). Our study demonstrates that IFN-γ reverses TGF-β impairment of maturation/MHC I presentation in infected DCs and activates bacterial elimination, predominantly by inducing iNOS/NO. Induced NO synthesis strongly affects bacterial growth and infectivity. Moreover, our studies hint that Coxiella-infected DCs might be able to protect themselves from mitotoxic NO by switching from oxidative phosphorylation to glycolysis, thus ensuring survival in self-defense against C. burnetii. Our results provide new insights into DC subversion by Coxiella and the IFN-γ-mediated targeting of C. burnetii during early steps in the innate immune response.
Keywords: Coxiella burnetii; immune subversion; major histocompatibility complex.
Conflict of interest statement
The authors declare no conflict of interest.
Figures
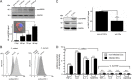
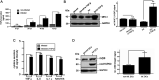
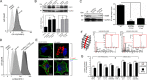
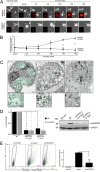
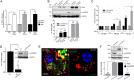
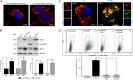
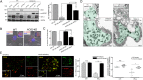
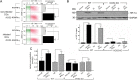
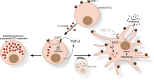
Similar articles
-
Both Major Histocompatibility Complex Class I (MHC-I) and MHC-II Molecules Are Required, while MHC-I Appears To Play a Critical Role in Host Defense against Primary Coxiella burnetii Infection.Infect Immun. 2018 Mar 22;86(4):e00602-17. doi: 10.1128/IAI.00602-17. Print 2018 Apr. Infect Immun. 2018. PMID: 29311245 Free PMC article.
-
Virulent Coxiella burnetii does not activate human dendritic cells: role of lipopolysaccharide as a shielding molecule.Proc Natl Acad Sci U S A. 2005 Jun 14;102(24):8722-7. doi: 10.1073/pnas.0501863102. Epub 2005 Jun 6. Proc Natl Acad Sci U S A. 2005. PMID: 15939879 Free PMC article.
-
Attenuated Coxiella burnetii phase II causes a febrile response in gamma interferon knockout and Toll-like receptor 2 knockout mice and protects against reinfection.Infect Immun. 2007 Dec;75(12):5845-58. doi: 10.1128/IAI.00901-07. Epub 2007 Sep 24. Infect Immun. 2007. PMID: 17893129 Free PMC article.
-
Recent Advances on the Innate Immune Response to Coxiella burnetii.Front Cell Infect Microbiol. 2021 Nov 2;11:754455. doi: 10.3389/fcimb.2021.754455. eCollection 2021. Front Cell Infect Microbiol. 2021. PMID: 34796128 Free PMC article. Review.
-
Immune response and Coxiella burnetii invasion.Adv Exp Med Biol. 2012;984:287-98. doi: 10.1007/978-94-007-4315-1_15. Adv Exp Med Biol. 2012. PMID: 22711638 Review.
Cited by
-
Q fever immunology: the quest for a safe and effective vaccine.NPJ Vaccines. 2023 Sep 7;8(1):133. doi: 10.1038/s41541-023-00727-6. NPJ Vaccines. 2023. PMID: 37679410 Free PMC article. Review.
-
Lysosomal trafficking regulator restricts intracellular growth of Coxiella burnetii by inhibiting the expansion of Coxiella-containing vacuole and upregulating nos2 expression.Front Cell Infect Microbiol. 2024 Jan 11;13:1336600. doi: 10.3389/fcimb.2023.1336600. eCollection 2023. Front Cell Infect Microbiol. 2024. PMID: 38282619 Free PMC article.
-
Beyond its preferential niche: Brucella abortus RNA down-modulates the IFN-γ-induced MHC-I expression in epithelial and endothelial cells.PLoS One. 2024 Jul 9;19(7):e0306429. doi: 10.1371/journal.pone.0306429. eCollection 2024. PLoS One. 2024. PMID: 38980867 Free PMC article.
References
Publication types
MeSH terms
Substances
LinkOut - more resources
Full Text Sources
Research Materials