miRNA-encapsulated abiotic materials and biovectors for cutaneous and oral wound healing: Biogenesis, mechanisms, and delivery nanocarriers
- PMID: 36684081
- PMCID: PMC9842058
- DOI: 10.1002/btm2.10343
miRNA-encapsulated abiotic materials and biovectors for cutaneous and oral wound healing: Biogenesis, mechanisms, and delivery nanocarriers
Abstract
MicroRNAs (miRNAs) as therapeutic agents have attracted increasing interest in the past decade owing to their significant effectiveness in treating a wide array of ailments. These polymerases II-derived noncoding RNAs act through post-transcriptional controlling of different proteins and their allied pathways. Like other areas of medicine, researchers have utilized miRNAs for managing acute and chronic wounds. The increase in the number of patients suffering from either under-healing or over-healing wound demonstrates the limited efficacy of the current wound healing strategies and dictates the demands for simpler approaches with greater efficacy. Various miRNA can be designed to induce pathway beneficial for wound healing. However, the proper design of miRNA and its delivery system for wound healing applications are still challenging due to their limited stability and intracellular delivery. Therefore, new miRNAs are required to be identified and their delivery strategy needs to be optimized. In this review, we discuss the diverse roles of miRNAs in various stages of wound healing and provide an insight on the most recent findings in the nanotechnology and biomaterials field, which might offer opportunities for the development of new strategies for this chronic condition. We also highlight the advances in biomaterials and delivery systems, emphasizing their challenges and resolutions for miRNA-based wound healing. We further review various biovectors (e.g., adenovirus and lentivirus) and abiotic materials such as organic and inorganic nanomaterials, along with dendrimers and scaffolds, as the delivery systems for miRNA-based wound healing. Finally, challenges and opportunities for translation of miRNA-based strategies into clinical applications are discussed.
Keywords: abiotic nanomaterials; chronic wounds; miRNA delivery; nanobiovectors; oral mucosa wound; viral and nonviral nanocarriers.
© 2022 The Authors. Bioengineering & Translational Medicine published by Wiley Periodicals LLC on behalf of American Institute of Chemical Engineers.
Conflict of interest statement
The authors declare no potential conflicts of interest.
Figures
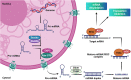
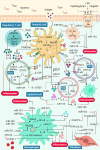
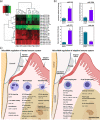
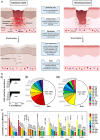
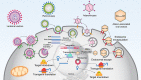
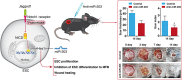
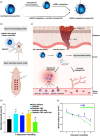
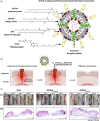
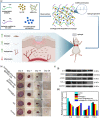
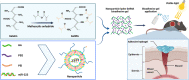
Similar articles
-
Extracellular vesicle-based biovectors in chronic wound healing: Biogenesis and delivery approaches.Mol Ther Nucleic Acids. 2023 May 9;32:822-840. doi: 10.1016/j.omtn.2023.05.002. eCollection 2023 Jun 13. Mol Ther Nucleic Acids. 2023. PMID: 37273778 Free PMC article. Review.
-
Nanomaterials-based Drug Delivery Approaches for Wound Healing.Curr Pharm Des. 2022;28(9):711-726. doi: 10.2174/1381612828666220328121211. Curr Pharm Des. 2022. PMID: 35345993 Review.
-
Nucleic acid-based therapeutics for dermal wound healing.Int J Biol Macromol. 2022 Nov 1;220:920-933. doi: 10.1016/j.ijbiomac.2022.08.099. Epub 2022 Aug 18. Int J Biol Macromol. 2022. PMID: 35987365 Review.
-
Development of microRNA-21 mimic nanocarriers for the treatment of cutaneous wounds.Theranostics. 2020 Feb 10;10(7):3240-3253. doi: 10.7150/thno.39870. eCollection 2020. Theranostics. 2020. PMID: 32194865 Free PMC article.
-
A Bioswitchable MiRNA Delivery System: Tetrahedral Framework DNA-Based miRNA Delivery System for Applications in Wound Healing.ACS Appl Mater Interfaces. 2024 Jul 3;16(26):33192-33204. doi: 10.1021/acsami.4c06460. Epub 2024 Jun 17. ACS Appl Mater Interfaces. 2024. PMID: 38885077
Cited by
-
Current advances in the development of microRNA-integrated tissue engineering strategies: a cornerstone of regenerative medicine.Front Bioeng Biotechnol. 2024 Oct 16;12:1484151. doi: 10.3389/fbioe.2024.1484151. eCollection 2024. Front Bioeng Biotechnol. 2024. PMID: 39479296 Free PMC article. Review.
-
Genetically Engineered Viral Vectors and Organic-Based Non-Viral Nanocarriers for Drug Delivery Applications.Adv Healthc Mater. 2022 Oct;11(20):e2201583. doi: 10.1002/adhm.202201583. Epub 2022 Aug 15. Adv Healthc Mater. 2022. PMID: 35916145 Free PMC article. Review.
-
Cell loaded hydrogel containing Ag-doped bioactive glass-ceramic nanoparticles as skin substitute: Antibacterial properties, immune response, and scarless cutaneous wound regeneration.Bioeng Transl Med. 2022 Aug 3;7(3):e10386. doi: 10.1002/btm2.10386. eCollection 2022 Sep. Bioeng Transl Med. 2022. PMID: 36176609 Free PMC article.
-
Encapsulation of Vitamin C by Glycerol-Derived Dendrimers, Their Interaction with Biomimetic Models of Stratum corneum and Their Cytotoxicity.Molecules. 2022 Nov 18;27(22):8022. doi: 10.3390/molecules27228022. Molecules. 2022. PMID: 36432124 Free PMC article.
-
Metal-Phenolic Networks for Chronic Wounds Therapy.Int J Nanomedicine. 2023 Nov 8;18:6425-6448. doi: 10.2147/IJN.S434535. eCollection 2023. Int J Nanomedicine. 2023. PMID: 38026522 Free PMC article. Review.
References
-
- Gupta S, Sagar S, Kisaka T, et al. Chronic wounds: magnitude, socioeconomic burden and consequences. Wounds Asia. 2021;4(1):8‐14.
-
- Posnett J, Franks P. The costs of skin breakdown and ulceration in the UK. In: Pownall M, ed. Skin Breakdown: The Silent Epidemic. The Smith and Nephew Foundation. 2007;6–12.
Publication types
LinkOut - more resources
Full Text Sources
Miscellaneous