Movement Protein Mediates Systemic Necrosis in Tomato Plants with Infection of Tomato Mosaic Virus
- PMID: 36680197
- PMCID: PMC9861833
- DOI: 10.3390/v15010157
Movement Protein Mediates Systemic Necrosis in Tomato Plants with Infection of Tomato Mosaic Virus
Abstract
The necrogenic strain N5 of tomato mosaic virus (ToMV-N5) causes systemic necrosis in tomato cultivar Hezuo903. In this work, we mapped the viral determinant responsible for the induction of systemic necrosis. By exchanging viral genes between N5 and a non-necrogenic strain S1, we found that movement protein (MP) was the determinant for the differential symptoms caused by both strains. Compared with S1 MP, N5 MP had an additional ability to increase virus accumulation, which was not due to its functions in viral cell-to-cell movement. Actually, N5 MP, but not S1 MP, was a weak RNA silencing suppressor, which assisted viral accumulation. Sequence alignment showed that both MPs differed by only three amino acid residues. Experiments with viruses having mutated MPs indicated that the residue isoleucine at position 170 in MP was the key site for MP to increase virus accumulation, but also was required for MP to induce systemic necrosis in virus-infected tomato plants. Collectively, the lethal necrosis caused by N5 is dependent on its MP protein that enhances virus accumulation via its RNA silencing suppressor activity, probably leading to systemic necrosis responses in tomato plants.
Keywords: RNA silencing suppressor; Solanum lycopersicum; movement protein; systemic necrosis; tomato mosaic virus.
Conflict of interest statement
The authors declare no conflict of interest.
Figures
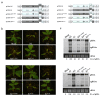
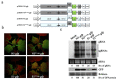
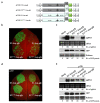
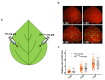
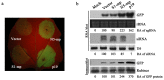
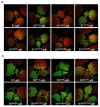
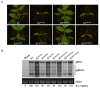
Similar articles
-
Nonsense mutations of replicase and movement protein genes contribute to the attenuation of an avirulent tomato mosaic virus.Virus Res. 2002 Aug;87(2):119-28. doi: 10.1016/s0168-1702(02)00025-4. Virus Res. 2002. PMID: 12191775
-
Identification of an amino acid residue required for differential recognition of a viral movement protein by the Tomato mosaic virus resistance gene Tm-2(2).J Plant Physiol. 2011 Jul 1;168(10):1142-5. doi: 10.1016/j.jplph.2011.01.002. Epub 2011 Feb 18. J Plant Physiol. 2011. PMID: 21310506
-
The Tomato Brown Rugose Fruit Virus Movement Protein Overcomes Tm-22 Resistance in Tomato While Attenuating Viral Transport.Mol Plant Microbe Interact. 2021 Sep;34(9):1024-1032. doi: 10.1094/MPMI-01-21-0023-R. Epub 2021 Oct 11. Mol Plant Microbe Interact. 2021. PMID: 33970669
-
Modulation of host plant immunity by Tobamovirus proteins.Ann Bot. 2017 Mar 1;119(5):737-747. doi: 10.1093/aob/mcw216. Ann Bot. 2017. PMID: 27941090 Free PMC article. Review.
-
Tomato brown rugose fruit virus: An emerging and rapidly spreading plant RNA virus that threatens tomato production worldwide.Mol Plant Pathol. 2022 Sep;23(9):1262-1277. doi: 10.1111/mpp.13229. Epub 2022 May 22. Mol Plant Pathol. 2022. PMID: 35598295 Free PMC article. Review.
Cited by
-
Role of Plant Virus Movement Proteins in Suppression of Host RNAi Defense.Int J Mol Sci. 2023 May 20;24(10):9049. doi: 10.3390/ijms24109049. Int J Mol Sci. 2023. PMID: 37240394 Free PMC article. Review.
References
-
- Nishikiori M., Dohi K., Mori M., Meshi T., Naito S., Ishikawa M. Membrane-bound tomato mosaic virus replication proteins participate in RNA synthesis and are associated with host proteins in a pattern distinct from those that are not membrane bound. J. Virol. 2006;80:8459–8468. doi: 10.1128/JVI.00545-06. - DOI - PMC - PubMed
Publication types
MeSH terms
Substances
Supplementary concepts
Grants and funding
LinkOut - more resources
Full Text Sources