First Evidence of the Protective Effects of 2-Pentadecyl-2-Oxazoline (PEA-OXA) in In Vitro Models of Acute Lung Injury
- PMID: 36671418
- PMCID: PMC9855419
- DOI: 10.3390/biom13010033
First Evidence of the Protective Effects of 2-Pentadecyl-2-Oxazoline (PEA-OXA) in In Vitro Models of Acute Lung Injury
Abstract
Acute respiratory distress syndrome (ARDS) is a serious inflammatory lung disorder and a complication of SARS-CoV-2 infection. In patients with severe SARS-CoV-2 infection, the transition to ARDS is principally due to the occurrence of a cytokine storm and an exacerbated inflammatory response. The effectiveness of ultra-micronized palmitoylethanolamide (PEA-um) during the earliest stage of COVID-19 has already been suggested. In this study, we evaluated its protective effects as well as the effectiveness of its congener, 2-pentadecyl-2-oxazoline (PEA-OXA), using in vitro models of acute lung injury. In detail, human lung epithelial cells (A549) activated by polyinosinic-polycytidylic acid (poly-(I:C)) or Transforming Growth Factor-beta (TGF-β) were treated with PEA-OXA or PEA. The release of IL-6 and the appearance of Epithelial-Mesenchymal Transition (EMT) were measured by ELISA and immunofluorescence assays, respectively. A possible mechanism of action for PEA-OXA and PEA was also investigated. Our results showed that both PEA-OXA and PEA were able to counteract poly-(I:C)-induced IL-6 release, as well as to revert TGF-β-induced EMT. In addition, PEA was able to produce an "entourage" effect on the levels of the two endocannabinoids AEA and 2-AG, while PEA-OXA only increased PEA endogenous levels, in poly-(I:C)-stimulated A549 cells. These results evidence for the first time the superiority of PEA-OXA over PEA in exerting protective effects and point to PEA-OXA as a new promising candidate in the management of acute lung injury.
Keywords: 2-pentadecyl-2-oxazoline; acute respiratory distress syndrome; anti-inflammatory; endocannabinoids; fibrosis; lung epithelial cells; palmitoylethanolamide.
Conflict of interest statement
A.S.M., M.A. and S.P. are employees of the Epitech Group SpA. S.P. and V.D. are co-inventors on patents on Adelmidrol and/or PEA, respectively, which are unrelated to the present study. The other authors declare no other conflict of interest.
Figures
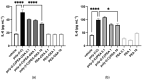
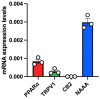
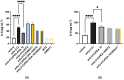
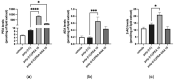
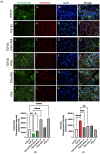
Similar articles
-
2-Pentadecyl-2-Oxazoline, the Oxazoline of Pea, Modulates Carrageenan-Induced Acute Inflammation.Front Pharmacol. 2017 May 30;8:308. doi: 10.3389/fphar.2017.00308. eCollection 2017. Front Pharmacol. 2017. PMID: 28611664 Free PMC article.
-
Management of Acute Lung Injury: Palmitoylethanolamide as a New Approach.Int J Mol Sci. 2021 May 24;22(11):5533. doi: 10.3390/ijms22115533. Int J Mol Sci. 2021. PMID: 34073872 Free PMC article.
-
Treatment With 2-Pentadecyl-2-Oxazoline Restores Mild Traumatic Brain Injury-Induced Sensorial and Neuropsychiatric Dysfunctions.Front Pharmacol. 2020 Feb 25;11:91. doi: 10.3389/fphar.2020.00091. eCollection 2020. Front Pharmacol. 2020. PMID: 32161542 Free PMC article.
-
Role of pirfenidone in TGF-β pathways and other inflammatory pathways in acute respiratory syndrome coronavirus 2 (SARS-Cov-2) infection: a theoretical perspective.Pharmacol Rep. 2021 Jun;73(3):712-727. doi: 10.1007/s43440-021-00255-x. Epub 2021 Apr 21. Pharmacol Rep. 2021. PMID: 33880743 Free PMC article. Review.
-
Micronized / ultramicronized palmitoylethanolamide (PEA) as natural neuroprotector against COVID-19 inflammation.Prostaglandins Other Lipid Mediat. 2021 Jun;154:106540. doi: 10.1016/j.prostaglandins.2021.106540. Epub 2021 Feb 23. Prostaglandins Other Lipid Mediat. 2021. PMID: 33636368 Free PMC article. Review.
Cited by
-
Circulating endocannabinoid levels in SARS-CoV-2 infection and their potential role in the inflammatory response.Sci Rep. 2024 Aug 22;14(1):19558. doi: 10.1038/s41598-024-70172-5. Sci Rep. 2024. PMID: 39174572 Free PMC article.
-
Methylation of TTC4 interaction with HSP70 inhibits pyroptosis in macrophages of sepsis-induced lung injury by NLRP3 inflammation.Am J Cancer Res. 2023 Nov 15;13(11):5122-5137. eCollection 2023. Am J Cancer Res. 2023. PMID: 38058818 Free PMC article.
-
SnRNA-Seq analysis reveals ten hub genes associated with alveolar epithelial cell injury during pulmonary acute respiratory distress syndrome.Heliyon. 2023 Jun 12;9(6):e17160. doi: 10.1016/j.heliyon.2023.e17160. eCollection 2023 Jun. Heliyon. 2023. PMID: 37389073 Free PMC article.
References
-
- Khodadadi H., Salles É.L., Jarrahi A., Chibane F., Costigliola V., Yu J.C., Vaibhav K., Hess D.C., Dhandapani K.M., Baban B. Cannabidiol Modulates Cytokine Storm in Acute Respiratory Distress Syndrome Induced by Simulated Viral Infection Using Synthetic RNA. Cannabi. annabinoid. Res. 2020;5:197–201. doi: 10.1089/can.2020.0043. - DOI - PMC - PubMed
MeSH terms
Substances
Grants and funding
LinkOut - more resources
Full Text Sources
Medical
Miscellaneous