Base editing screens map mutations affecting interferon-γ signaling in cancer
- PMID: 36669486
- PMCID: PMC9942875
- DOI: 10.1016/j.ccell.2022.12.009
Base editing screens map mutations affecting interferon-γ signaling in cancer
Abstract
Interferon-γ (IFN-γ) signaling mediates host responses to infection, inflammation and anti-tumor immunity. Mutations in the IFN-γ signaling pathway cause immunological disorders, hematological malignancies, and resistance to immune checkpoint blockade (ICB) in cancer; however, the function of most clinically observed variants remains unknown. Here, we systematically investigate the genetic determinants of IFN-γ response in colorectal cancer cells using CRISPR-Cas9 screens and base editing mutagenesis. Deep mutagenesis of JAK1 with cytidine and adenine base editors, combined with pathway-wide screens, reveal loss-of-function and gain-of-function mutations, including causal variants in hematological malignancies and mutations detected in patients refractory to ICB. We functionally validate variants of uncertain significance in primary tumor organoids, where engineering missense mutations in JAK1 enhanced or reduced sensitivity to autologous tumor-reactive T cells. We identify more than 300 predicted missense mutations altering IFN-γ pathway activity, generating a valuable resource for interpreting gene variant function.
Keywords: IFN-γ signaling; base editing; cancer genetics; cancer immunotherapy; drug resistance; functional genomics; gene editing; interferon gamma; variants of uncertain significance.
Copyright © 2023 The Authors. Published by Elsevier Inc. All rights reserved.
Conflict of interest statement
Declaration of interests M.J.G. has received research grants from AstraZeneca, GlaxoSmithKline, and Astex Pharmaceuticals, and is a founder and advisor for Mosaic Therapeutics.
Figures
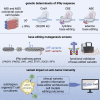
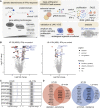
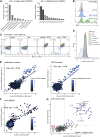
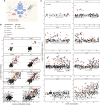

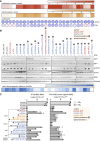
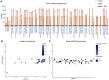
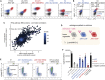
Similar articles
-
Identification of pathogenic variants in cancer genes using base editing screens with editing efficiency correction.Genome Biol. 2021 Mar 10;22(1):80. doi: 10.1186/s13059-021-02305-2. Genome Biol. 2021. PMID: 33691754 Free PMC article.
-
Mutation patterns in genes encoding interferon signaling and antigen presentation: A pan-cancer survey with implications for the use of immune checkpoint inhibitors.Genes Chromosomes Cancer. 2017 Aug;56(8):651-659. doi: 10.1002/gcc.22468. Epub 2017 May 31. Genes Chromosomes Cancer. 2017. PMID: 28466543
-
Precise in vivo functional analysis of DNA variants with base editing using ACEofBASEs target prediction.Elife. 2022 Apr 4;11:e72124. doi: 10.7554/eLife.72124. Elife. 2022. PMID: 35373735 Free PMC article.
-
IFNγ signaling integrity in colorectal cancer immunity and immunotherapy.Cell Mol Immunol. 2022 Jan;19(1):23-32. doi: 10.1038/s41423-021-00735-3. Epub 2021 Aug 12. Cell Mol Immunol. 2022. PMID: 34385592 Free PMC article. Review.
-
Immunotherapy to get on point with base editing.Drug Discov Today. 2021 Oct;26(10):2350-2357. doi: 10.1016/j.drudis.2021.04.003. Epub 2021 Apr 20. Drug Discov Today. 2021. PMID: 33857616 Review.
Cited by
-
Mapping drug resistance variants in cancer, one base at a time.Nat Genet. 2024 Oct 25. doi: 10.1038/s41588-024-01976-4. Online ahead of print. Nat Genet. 2024. PMID: 39455894 No abstract available.
-
Base editing screens define the genetic landscape of cancer drug resistance mechanisms.Nat Genet. 2024 Oct 18. doi: 10.1038/s41588-024-01948-8. Online ahead of print. Nat Genet. 2024. PMID: 39424923
-
Strategies for improving the genome-editing efficiency of class 2 CRISPR/Cas system.Heliyon. 2024 Sep 27;10(19):e38588. doi: 10.1016/j.heliyon.2024.e38588. eCollection 2024 Oct 15. Heliyon. 2024. PMID: 39397905 Free PMC article. Review.
-
Differential expression of ST6GALNAC1 and ST6GALNAC2 and their clinical relevance to colorectal cancer progression.PLoS One. 2024 Sep 30;19(9):e0311212. doi: 10.1371/journal.pone.0311212. eCollection 2024. PLoS One. 2024. PMID: 39348343 Free PMC article.
-
Integrative analysis of the immunological significances of guanylate binding protein family genes in microsatellite stability colorectal cancer.Heliyon. 2024 Sep 10;10(18):e37741. doi: 10.1016/j.heliyon.2024.e37741. eCollection 2024 Sep 30. Heliyon. 2024. PMID: 39315131 Free PMC article.
References
-
- Lupardus P.J., Ultsch M., Wallweber H., Bir Kohli P., Johnson A.R., Eigenbrot C. Structure of the pseudokinase-kinase domains from protein kinase TYK2 reveals a mechanism for Janus kinase (JAK) autoinhibition. Proc. Natl. Acad. Sci. USA. 2014;111:8025–8030. doi: 10.1073/pnas.1401180111. - DOI - PMC - PubMed
-
- Zaretsky J.M., Garcia-Diaz A., Shin D.S., Escuin-Ordinas H., Hugo W., Hu-Lieskovan S., Torrejon D.Y., Abril-Rodriguez G., Sandoval S., Barthly L., et al. Mutations associated with acquired resistance to PD-1 blockade in melanoma. N. Engl. J. Med. 2016;375:819–829. doi: 10.1056/NEJMoa1604958. - DOI - PMC - PubMed
Publication types
MeSH terms
Substances
Grants and funding
LinkOut - more resources
Full Text Sources
Research Materials
Miscellaneous