The cellular pathways that maintain the quality control and transport of diverse potassium channels
- PMID: 36638864
- PMCID: PMC9908860
- DOI: 10.1016/j.bbagrm.2023.194908
The cellular pathways that maintain the quality control and transport of diverse potassium channels
Abstract
Potassium channels are multi-subunit transmembrane proteins that permit the selective passage of potassium and play fundamental roles in physiological processes, such as action potentials in the nervous system and organismal salt and water homeostasis, which is mediated by the kidney. Like all ion channels, newly translated potassium channels enter the endoplasmic reticulum (ER) and undergo the error-prone process of acquiring post-translational modifications, folding into their native conformations, assembling with other subunits, and trafficking through the secretory pathway to reach their final destinations, most commonly the plasma membrane. Disruptions in these processes can result in detrimental consequences, including various human diseases. Thus, multiple quality control checkpoints evolved to guide potassium channels through the secretory pathway and clear potentially toxic, aggregation-prone misfolded species. We will summarize current knowledge on the mechanisms underlying potassium channel quality control in the secretory pathway, highlight diseases associated with channel misfolding, and suggest potential therapeutic routes.
Keywords: Endocytosis; Endoplasmic reticulum associated degradation (ERAD); Endosomal sorting; Molecular chaperones; Plasma membrane quality control; Protein quality control.
Copyright © 2023 Elsevier B.V. All rights reserved.
Conflict of interest statement
Declaration of competing interest The authors declare no conflicts of interest.
Figures
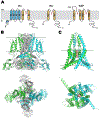
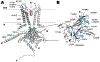
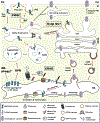
Similar articles
-
Disposing of misfolded ER proteins: A troubled substrate's way out of the ER.Mol Cell Endocrinol. 2020 Jan 15;500:110630. doi: 10.1016/j.mce.2019.110630. Epub 2019 Oct 24. Mol Cell Endocrinol. 2020. PMID: 31669350 Free PMC article. Review.
-
How Is the Fidelity of Proteins Ensured in Terms of Both Quality and Quantity at the Endoplasmic Reticulum? Mechanistic Insights into E3 Ubiquitin Ligases.Int J Mol Sci. 2021 Feb 19;22(4):2078. doi: 10.3390/ijms22042078. Int J Mol Sci. 2021. PMID: 33669844 Free PMC article. Review.
-
Roles of molecular chaperones in endoplasmic reticulum (ER) quality control and ER-associated degradation (ERAD).J Biochem. 2005 May;137(5):551-5. doi: 10.1093/jb/mvi068. J Biochem. 2005. PMID: 15944407 Review.
-
The glycan-dependent ERAD machinery degrades topologically diverse misfolded proteins.Plant J. 2018 Apr;94(2):246-259. doi: 10.1111/tpj.13851. Epub 2018 Mar 14. Plant J. 2018. PMID: 29396984 Free PMC article.
-
Inhibiting endoplasmic reticulum (ER)-associated degradation of misfolded Yor1p does not permit ER export despite the presence of a diacidic sorting signal.Mol Biol Cell. 2007 Sep;18(9):3398-413. doi: 10.1091/mbc.e07-01-0046. Epub 2007 Jul 5. Mol Biol Cell. 2007. PMID: 17615300 Free PMC article.
Cited by
-
Genome mining yields putative disease-associated ROMK variants with distinct defects.PLoS Genet. 2023 Nov 13;19(11):e1011051. doi: 10.1371/journal.pgen.1011051. eCollection 2023 Nov. PLoS Genet. 2023. PMID: 37956218 Free PMC article.
-
Identification of key potassium channel genes of temporal lobe epilepsy by bioinformatics analyses and experimental verification.Front Neurol. 2023 Jul 7;14:1175007. doi: 10.3389/fneur.2023.1175007. eCollection 2023. Front Neurol. 2023. PMID: 37483435 Free PMC article.
-
Extracellular histones promote TWIK2-dependent potassium efflux and associated NLRP3 activation in alveolar macrophages during sepsis-induced lung injury.Inflamm Res. 2024 Jul;73(7):1137-1155. doi: 10.1007/s00011-024-01888-3. Epub 2024 May 11. Inflamm Res. 2024. PMID: 38733398
References
Publication types
MeSH terms
Substances
Grants and funding
LinkOut - more resources
Full Text Sources