Forages and Pastures Symposium: revisiting mechanisms, methods, and models for altering forage cell wall utilization for ruminants
- PMID: 36617721
- PMCID: PMC10313096
- DOI: 10.1093/jas/skad009
Forages and Pastures Symposium: revisiting mechanisms, methods, and models for altering forage cell wall utilization for ruminants
Abstract
Several ruminant animals rely almost exclusively on the complex polysaccharide matrix from the plant cell wall (CW) as their primary energy source via volatile fatty acids produced through ruminal and some hindgut fermentation processes. The CW contains different types and proportions of polysaccharides, proteins, phenolic compounds, and minerals in their macromolecular structure that influence the rate and extent of fiber digestion and selective retention of particulate matter due to its physical characteristics (buoyancy and comminuting) in the reticulorumen. The biosynthetic formation of the CW dictates possible manipulation mechanisms (targeted plant and microbes selection) and processing methods (physical, chemical, microbial, and enzymatic treatments and the use of genetically engineered bacteria) to increase its digestibility, leading to better utilization of the CW by the ruminant animal and hopefully lower the contribution of ruminants' greenhouse gas emissions. Early studies on lignin biosynthesis have led to more advanced studies focusing on replacing traditional monolignols with homopolymers that are easier to deconstruct or degrade. Concurrently, laboratory methods must be developed, evaluated, and modified to accurately reflect the digestibility and nutritive value of CW brought about by modern manipulation mechanisms or processing methods. However, the laboratory methods must also be reliable, precise, feasible, trivial, easy to implement, and cost-effective, but at the same time environmentally friendly and aware. For instance, although the acid detergent lignin has been demonstrated to behave uniformly as a nutritional entity, its chemical determination and association with carbohydrates still lack consensus. Spectroscopy (near-infrared and Raman) and in vitro gas production techniques have been adopted to assess plant chemical composition and nutritive value, but an incomplete understanding of the impacts caused by disrupting the CW for sample processing still exists. Different variations of multicompartmental and time- and age-dependent mathematical models have been proposed to determine the ruminal rates of degradation and passage of fiber. However, low-quality and incomplete data due to inconsistent marker results used to determine passage rates and transit time of fiber in the gastrointestinal tract have hindered advancements and adoptions of the next generation of computer models to understand ruminal fiber degradation.
Keywords: assessment; composition; fiber; model; nutritive value; prediction.
Plain language summary
The underlying principles of forage cell wall utilization by ruminants have been known for over 50 years, but a significant amount of knowledge of the structure and synthesis of critical components of the plant cell wall, mechanisms and methods to alter its digestibility, and assessment techniques to quantify its components as well as their fermentability has been accumulated in the last 30 years. Such knowledge has even allowed us to make recommendations about the importance of fiber in the diet to improve animal performance and welfare. For instance, some industries (especially the paper mill and biofuels) have attained significant advancements toward modifying plant lignin (a critical component of the cell wall that reduces fermentability) and lignin-degrading microorganisms that could assist the animal nutrition community in increasing the digestibility of forage cell wall without further pretreatment. There are many techniques and technologies available to increase cell wall digestibility and, consequently, animal productivity. However, each has potential and limitations, and when used alone, it may not yield the best outcome. From a ruminant nutrition perspective, combining such techniques and technologies with the next generation of mathematical models seems more likely to yield significant improvements in forage cell wall digestibility.
© The Author(s) 2023. Published by Oxford University Press on behalf of the American Society of Animal Science.
Conflict of interest statement
The authors declare no perceived conflict of interest.
Figures
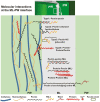
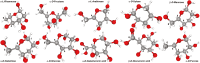
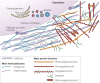
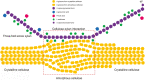
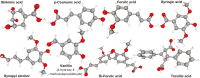
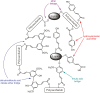
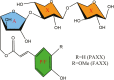
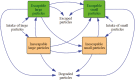
Similar articles
-
Forages and pastures symposium: an update on in vitro and in situ experimental techniques for approximation of ruminal fiber degradation.J Anim Sci. 2023 Jan 3;101:skad097. doi: 10.1093/jas/skad097. J Anim Sci. 2023. PMID: 37403237 Free PMC article.
-
Forages and pastures symposium: forage biodegradation: advances in ruminal microbial ecology.J Anim Sci. 2023 Jan 3;101:skad178. doi: 10.1093/jas/skad178. J Anim Sci. 2023. PMID: 37257501 Free PMC article.
-
Characteristics of plant cell walls affecting intake and digestibility of forages by ruminants.J Anim Sci. 1995 Sep;73(9):2774-90. doi: 10.2527/1995.7392774x. J Anim Sci. 1995. PMID: 8582870 Review.
-
Effect of lignin linkages with other plant cell wall components on in vitro and in vivo neutral detergent fiber digestibility and rate of digestion of grass forages.J Dairy Sci. 2017 Oct;100(10):8119-8131. doi: 10.3168/jds.2016-12364. Epub 2017 Aug 2. J Dairy Sci. 2017. PMID: 28780096
-
Analysis of forage fiber and cell walls in ruminant nutrition.J Nutr. 1997 May;127(5 Suppl):810S-813S. doi: 10.1093/jn/127.5.810S. J Nutr. 1997. PMID: 9164242 Review.
Cited by
-
Conceptualization and implementation of the Fiber Utilization and Cell Wall Constituents Symposium.J Anim Sci. 2023 Jan 3;101:skad200. doi: 10.1093/jas/skad200. J Anim Sci. 2023. PMID: 37399291 Free PMC article. No abstract available.
-
Forages and pastures symposium: an update on in vitro and in situ experimental techniques for approximation of ruminal fiber degradation.J Anim Sci. 2023 Jan 3;101:skad097. doi: 10.1093/jas/skad097. J Anim Sci. 2023. PMID: 37403237 Free PMC article.
-
Forages and pastures symposium: forage biodegradation: advances in ruminal microbial ecology.J Anim Sci. 2023 Jan 3;101:skad178. doi: 10.1093/jas/skad178. J Anim Sci. 2023. PMID: 37257501 Free PMC article.
References
-
- Aderinboye, R. Y., Akinlolu A. O., Adeleke M. A., Najeem G. O., Ojo V. O. A., Isah O. A., and Babayemi O. J.. . 2016. In vitro gas production and dry matter degradation of four browse leaves using cattle, sheep and goat inocula. Slovak J. Anim. Sci. 49(1):32–43.
-
- Akin, D. E. 1989. Histological and physical factors affecting digestibility of forages. Agron. J. 81:17–25. doi:10.2134/agronj1989.00021962008100010004x - DOI
MeSH terms
Substances
LinkOut - more resources
Full Text Sources