Proteolytic Degradation Is a Major Contributor to Bioprosthetic Heart Valve Failure
- PMID: 36565196
- PMCID: PMC9973599
- DOI: 10.1161/JAHA.122.028215
Proteolytic Degradation Is a Major Contributor to Bioprosthetic Heart Valve Failure
Abstract
Background Whereas the risk factors for structural valve degeneration (SVD) of glutaraldehyde-treated bioprosthetic heart valves (BHVs) are well studied, those responsible for the failure of BHVs fixed with alternative next-generation chemicals remain largely unknown. This study aimed to investigate the reasons behind the development of SVD in ethylene glycol diglycidyl ether-treated BHVs. Methods and Results Ten ethylene glycol diglycidyl ether-treated BHVs excised because of SVD, and 5 calcified aortic valves (AVs) replaced with BHVs because of calcific AV disease were collected and their proteomic profile was deciphered. Then, BHVs and AVs were interrogated for immune cell infiltration, microbial contamination, distribution of matrix-degrading enzymes and their tissue inhibitors, lipid deposition, and calcification. In contrast with dysfunctional AVs, failing BHVs suffered from complement-driven neutrophil invasion, excessive proteolysis, unwanted coagulation, and lipid deposition. Neutrophil infiltration was triggered by an asymptomatic bacterial colonization of the prosthetic tissue. Neutrophil elastase, myeloblastin/proteinase 3, cathepsin G, and matrix metalloproteinases (MMPs; neutrophil-derived MMP-8 and plasma-derived MMP-9), were significantly overexpressed, while tissue inhibitors of metalloproteinases 1/2 were downregulated in the BHVs as compared with AVs, together indicative of unbalanced proteolysis in the failing BHVs. As opposed to other proteases, MMP-9 was mostly expressed in the disorganized prosthetic extracellular matrix, suggesting plasma-derived proteases as the primary culprit of SVD in ethylene glycol diglycidyl ether-treated BHVs. Hence, hemodynamic stress and progressive accumulation of proteases led to the extracellular matrix degeneration and dystrophic calcification, ultimately resulting in SVD. Conclusions Neutrophil- and plasma-derived proteases are responsible for the loss of BHV mechanical competence and need to be thwarted to prevent SVD.
Keywords: bacterial invasion; bioprosthetic heart valves; matrix metalloproteinases; neutrophil infiltration; structural valve degeneration.
Figures
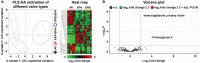
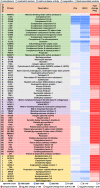
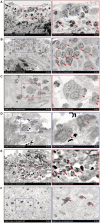
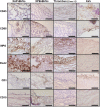
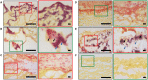
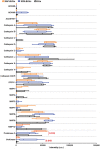
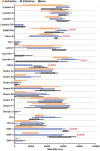
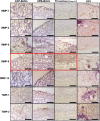
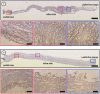
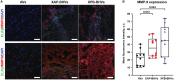
Similar articles
-
[Screening analysis of proteolytic enzymes and their inhibitors in the leaflets of epoxy-treated bioprosthetic heart valves explanted due to dysfunction].Biomed Khim. 2022 Jan;68(1):68-75. doi: 10.18097/PBMC20226801068. Biomed Khim. 2022. PMID: 35221298 Russian.
-
A universal strategy for the construction of polymer brush hybrid non-glutaraldehyde heart valves with robust anti-biological contamination performance and improved endothelialization potential.Acta Biomater. 2023 Apr 1;160:87-97. doi: 10.1016/j.actbio.2023.02.009. Epub 2023 Feb 21. Acta Biomater. 2023. PMID: 36812953
-
Reducing immunoreactivity of porcine bioprosthetic heart valves by genetically-deleting three major glycan antigens, GGTA1/β4GalNT2/CMAH.Acta Biomater. 2018 May;72:196-205. doi: 10.1016/j.actbio.2018.03.055. Epub 2018 Apr 7. Acta Biomater. 2018. PMID: 29631050
-
Degeneration of Bioprosthetic Heart Valves: Update 2020.J Am Heart Assoc. 2020 Oct 20;9(19):e018506. doi: 10.1161/JAHA.120.018506. Epub 2020 Sep 21. J Am Heart Assoc. 2020. PMID: 32954917 Free PMC article. Review.
-
Noncalcific Mechanisms of Bioprosthetic Structural Valve Degeneration.J Am Heart Assoc. 2021 Feb 2;10(3):e018921. doi: 10.1161/JAHA.120.018921. Epub 2021 Jan 26. J Am Heart Assoc. 2021. PMID: 33494616 Free PMC article. Review.
Cited by
-
Embedding and Backscattered Scanning Electron Microscopy (EM-BSEM) Is Preferential over Immunophenotyping in Relation to Bioprosthetic Heart Valves.Int J Mol Sci. 2023 Sep 2;24(17):13602. doi: 10.3390/ijms241713602. Int J Mol Sci. 2023. PMID: 37686408 Free PMC article.
-
ML-driven segmentation of microvascular features during histological examination of tissue-engineered vascular grafts.Front Bioeng Biotechnol. 2024 Jun 26;12:1411680. doi: 10.3389/fbioe.2024.1411680. eCollection 2024. Front Bioeng Biotechnol. 2024. PMID: 38988863 Free PMC article.
References
-
- Otto CM, Nishimura RA, Bonow RO, Carabello BA, Erwin JP III, Gentile F, Jneid H, Krieger EV, Mack M, McLeod C, et al. 2020 ACC/AHA guideline for the management of patients with valvular heart disease: a report of the American College of Cardiology/American Heart Association joint committee on clinical practice guidelines. Circulation. 2021;143:e72–e227. doi: 10.1161/CIR.0000000000000923 - DOI - PubMed
Publication types
MeSH terms
Substances
LinkOut - more resources
Full Text Sources
Medical
Research Materials
Miscellaneous