Target Metabolites to Slow Down Progression of Amyotrophic Lateral Sclerosis in Mice
- PMID: 36557291
- PMCID: PMC9784240
- DOI: 10.3390/metabo12121253
Target Metabolites to Slow Down Progression of Amyotrophic Lateral Sclerosis in Mice
Abstract
Microbial metabolites affect the neuron system and muscle cell functions. Amyotrophic lateral sclerosis (ALS) is a multifactorial neuromuscular disease. Our previous study has demonstrated elevated intestinal inflammation and dysfunction of the microbiome in patients with ALS and an ALS mouse model (human-SOD1G93A transgenic mice). However, the metabolites in ALS progression are unknown. Using an unbiased global metabolomic measurement and targeted measurement, we investigated the longitudinal changes of fecal metabolites in SOD1G93A mice over the course of 13 weeks. We further compared the changes of metabolites and inflammatory response in age-matched wild-type (WT) and SOD1G93A mice treated with the bacterial product butyrate. We found changes in carbohydrate levels, amino acid metabolism, and the formation of gamma-glutamyl amino acids. Shifts in several microbially contributed catabolites of aromatic amino acids agree with butyrate-induced changes in the composition of the gut microbiome. Declines in gamma-glutamyl amino acids in feces may stem from differential expression of gamma-glutamyltransferase (GGT) in response to butyrate administration. Due to the signaling nature of amino acid-derived metabolites, these changes indicate changes in inflammation, e.g., histamine, and contribute to differences in systemic levels of neurotransmitters, e.g., γ-Aminobutyric acid (GABA) and glutamate. Butyrate treatment was able to restore some of the healthy metabolites in ALS mice. Moreover, microglia in the spinal cord were measured by IBA1 staining. Butyrate treatment significantly suppressed the IBA1 level in the SOD1G93A mice. Serum IL-17 and LPS were significantly reduced in the butyrate-treated SOD1G93A mice. We have demonstrated an inter-organ communications link among microbial metabolites, neuroactive metabolites from the gut, and inflammation in ALS progression. The study supports the potential to use metabolites as ALS hallmarks and for treatment.
Keywords: ALS; CNS; FALS; SALS; dysbiosis; immunity; inflammation; longitudinal analysis; metabolites; neuromuscular disease; protein aggregation.
Conflict of interest statement
The authors declare no conflict of interest.
Figures
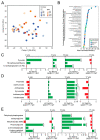
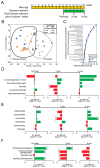
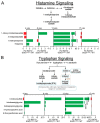
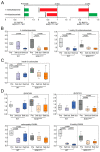
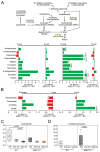
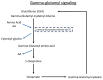
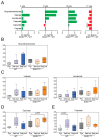
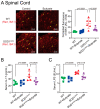
Similar articles
-
Knocking down metabotropic glutamate receptor 1 improves survival and disease progression in the SOD1(G93A) mouse model of amyotrophic lateral sclerosis.Neurobiol Dis. 2014 Apr;64:48-59. doi: 10.1016/j.nbd.2013.11.006. Epub 2013 Dec 19. Neurobiol Dis. 2014. PMID: 24361555
-
Microglia RAGE exacerbates the progression of neurodegeneration within the SOD1G93A murine model of amyotrophic lateral sclerosis in a sex-dependent manner.J Neuroinflammation. 2021 Jun 15;18(1):139. doi: 10.1186/s12974-021-02191-2. J Neuroinflammation. 2021. PMID: 34130712 Free PMC article.
-
Spinal Cord Metabolic Signatures in Models of Fast- and Slow-Progressing SOD1G93A Amyotrophic Lateral Sclerosis.Front Neurosci. 2019 Dec 10;13:1276. doi: 10.3389/fnins.2019.01276. eCollection 2019. Front Neurosci. 2019. PMID: 31920474 Free PMC article.
-
Long-term interleukin-33 treatment delays disease onset and alleviates astrocytic activation in a transgenic mouse model of amyotrophic lateral sclerosis.IBRO Rep. 2019 Jan 11;6:74-86. doi: 10.1016/j.ibror.2019.01.005. eCollection 2019 Jun. IBRO Rep. 2019. PMID: 30705990 Free PMC article.
-
In-vivo effects of knocking-down metabotropic glutamate receptor 5 in the SOD1G93A mouse model of amyotrophic lateral sclerosis.Neuropharmacology. 2017 Sep 1;123:433-445. doi: 10.1016/j.neuropharm.2017.06.020. Epub 2017 Jun 21. Neuropharmacology. 2017. PMID: 28645622
Cited by
-
Intestinal Barrier Function and Neurodegenerative Disease.CNS Neurol Disord Drug Targets. 2024;23(9):1134-1142. doi: 10.2174/0118715273264097231116103948. CNS Neurol Disord Drug Targets. 2024. PMID: 38008941 Review.
-
Gut Microbial Metabolome and Dysbiosis in Neurodegenerative Diseases: Psychobiotics and Fecal Microbiota Transplantation as a Therapeutic Approach-A Comprehensive Narrative Review.Int J Mol Sci. 2023 Aug 27;24(17):13294. doi: 10.3390/ijms241713294. Int J Mol Sci. 2023. PMID: 37686104 Free PMC article. Review.
-
Brain-Gut-Microbiota Axis in Amyotrophic Lateral Sclerosis: A Historical Overview and Future Directions.Aging Dis. 2024 Feb 1;15(1):74-95. doi: 10.14336/AD.2023.0524. Aging Dis. 2024. PMID: 37307822 Free PMC article. Review.
-
Metabolites and micronutrition in modulating amyotrophic lateral sclerosis.Neural Regen Res. 2024 Jun 1;19(6):1183-1184. doi: 10.4103/1673-5374.385861. Epub 2023 Sep 22. Neural Regen Res. 2024. PMID: 37905855 Free PMC article. No abstract available.
-
Gut-Modulating Agents and Amyotrophic Lateral Sclerosis: Current Evidence and Future Perspectives.Nutrients. 2024 Feb 21;16(5):590. doi: 10.3390/nu16050590. Nutrients. 2024. PMID: 38474719 Free PMC article. Review.
References
Grants and funding
LinkOut - more resources
Full Text Sources
Molecular Biology Databases
Miscellaneous