Visualizing the Genome: Experimental Approaches for Live-Cell Chromatin Imaging
- PMID: 36552850
- PMCID: PMC9776900
- DOI: 10.3390/cells11244086
Visualizing the Genome: Experimental Approaches for Live-Cell Chromatin Imaging
Abstract
Over the years, our vision of the genome has changed from a linear molecule to that of a complex 3D structure that follows specific patterns and possesses a hierarchical organization. Currently, genomics is becoming "four-dimensional": our attention is increasingly focused on the study of chromatin dynamics over time, in the fourth dimension. Recent methods for visualizing the movements of chromatin loci in living cells by targeting fluorescent proteins can be divided into two groups. The first group requires the insertion of a special sequence into the locus of interest, to which proteins that recognize the sequence are recruited (e.g., FROS and ParB-INT methods). In the methods of the second approach, "programmed" proteins are targeted to the locus of interest (i.e., systems based on CRISPR/Cas, TALE, and zinc finger proteins). In the present review, we discuss these approaches, examine their strengths and weaknesses, and identify the key scientific problems that can be studied using these methods.
Keywords: 3D genome; CRISPR imaging; FROS; ParB-INT; TALE imaging; chromatin dynamics; chromatin visualization; live-cell imaging; zinc finger imaging.
Conflict of interest statement
The authors declare no conflict of interest.
Figures
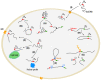
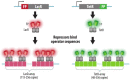
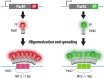
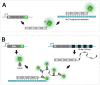
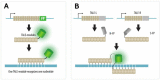
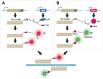
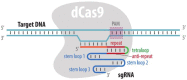
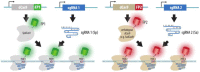
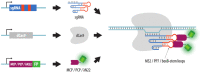
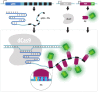
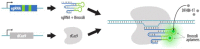
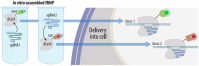
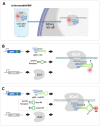
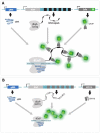
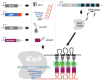
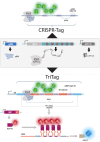
Similar articles
-
Live-cell imaging of chromatin contacts opens a new window into chromatin dynamics.Epigenetics Chromatin. 2023 Jun 23;16(1):27. doi: 10.1186/s13072-023-00503-9. Epigenetics Chromatin. 2023. PMID: 37349773 Free PMC article. Review.
-
Visualizing Live Chromatin Dynamics through CRISPR-Based Imaging Techniques.Mol Cells. 2021 Sep 30;44(9):627-636. doi: 10.14348/molcells.2021.2254. Mol Cells. 2021. PMID: 34588320 Free PMC article.
-
Application and prospects of CRISPR/Cas9-based methods to trace defined genomic sequences in living and fixed plant cells.Chromosome Res. 2020 Mar;28(1):7-17. doi: 10.1007/s10577-019-09622-0. Epub 2019 Dec 3. Chromosome Res. 2020. PMID: 31792795 Review.
-
Progress and Challenges for Live-cell Imaging of Genomic Loci Using CRISPR-based Platforms.Genomics Proteomics Bioinformatics. 2019 Apr;17(2):119-128. doi: 10.1016/j.gpb.2018.10.001. Epub 2019 Jan 30. Genomics Proteomics Bioinformatics. 2019. PMID: 30710789 Free PMC article. Review.
-
Visualizing looping of two endogenous genomic loci using synthetic zinc-finger proteins with anti-FLAG and anti-HA frankenbodies in living cells.Genes Cells. 2021 Nov;26(11):905-926. doi: 10.1111/gtc.12893. Epub 2021 Sep 20. Genes Cells. 2021. PMID: 34465007 Free PMC article.
Cited by
-
Live-cell imaging of chromatin contacts opens a new window into chromatin dynamics.Epigenetics Chromatin. 2023 Jun 23;16(1):27. doi: 10.1186/s13072-023-00503-9. Epigenetics Chromatin. 2023. PMID: 37349773 Free PMC article. Review.
-
CRISPR/Cas-Based Techniques for Live-Cell Imaging and Bioanalysis.Int J Mol Sci. 2023 Aug 30;24(17):13447. doi: 10.3390/ijms241713447. Int J Mol Sci. 2023. PMID: 37686249 Free PMC article. Review.
-
Opticool: Cutting-edge transgenic optical tools.PLoS Genet. 2024 Mar 22;20(3):e1011208. doi: 10.1371/journal.pgen.1011208. eCollection 2024 Mar. PLoS Genet. 2024. PMID: 38517915 Free PMC article. Review.
-
A Comparison of Two Versions of the CRISPR-Sirius System for the Live-Cell Visualization of the Borders of Topologically Associating Domains.Cells. 2024 Aug 27;13(17):1440. doi: 10.3390/cells13171440. Cells. 2024. PMID: 39273012 Free PMC article.
-
Su(H) Modulates Enhancer Transcriptional Bursting in Prelude to Gastrulation.Cells. 2024 Oct 24;13(21):1759. doi: 10.3390/cells13211759. Cells. 2024. PMID: 39513866 Free PMC article.
References
-
- Cabal G.G., Genovesio A., Rodriguez-Navarro S., Zimmer C., Gadal O., Lesne A., Buc H., Feuerbach-Fournier F., Olivo-Marin J.C., Hurt E.C., et al. SAGA interacting factors confine sub-diffusion of transcribed genes to the nuclear envelope. Nature. 2006;441:770–773. doi: 10.1038/nature04752. - DOI - PubMed
Publication types
MeSH terms
Substances
Grants and funding
LinkOut - more resources
Full Text Sources