Inheritance of paternal DNA damage by histone-mediated repair restriction
- PMID: 36544019
- PMCID: PMC9834056
- DOI: 10.1038/s41586-022-05544-w
Inheritance of paternal DNA damage by histone-mediated repair restriction
Abstract
How paternal exposure to ionizing radiation affects genetic inheritance and disease risk in the offspring has been a long-standing question in radiation biology. In humans, nearly 80% of transmitted mutations arise in the paternal germline1, but the transgenerational effects of ionizing radiation exposure has remained controversial and the mechanisms are unknown. Here we show that in sex-separated Caenorhabditis elegans strains, paternal, but not maternal, exposure to ionizing radiation leads to transgenerational embryonic lethality. The offspring of irradiated males displayed various genome instability phenotypes, including DNA fragmentation, chromosomal rearrangement and aneuploidy. Paternal DNA double strand breaks were repaired by maternally provided error-prone polymerase theta-mediated end joining. Mechanistically, we show that depletion of an orthologue of human histone H1.0, HIS-24, or the heterochromatin protein HPL-1, could significantly reverse the transgenerational embryonic lethality. Removal of HIS-24 or HPL-1 reduced histone 3 lysine 9 dimethylation and enabled error-free homologous recombination repair in the germline of the F1 generation from ionizing radiation-treated P0 males, consequently improving the viability of the F2 generation. This work establishes the mechanistic underpinnings of the heritable consequences of paternal radiation exposure on the health of offspring, which may lead to congenital disorders and cancer in humans.
© 2022. The Author(s).
Conflict of interest statement
B.S. is a co-founder of Agevio Therapeutics.
Figures
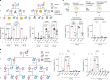
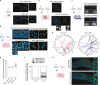
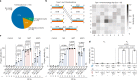
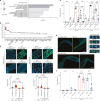
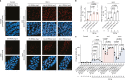
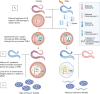
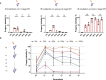
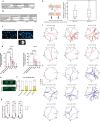
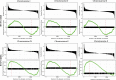
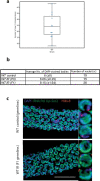
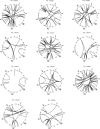
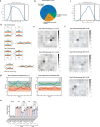
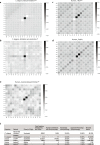
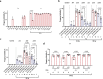
Comment in
-
A mechanism for inheriting radiation-induced DNA damage.Nature. 2023 Jan;613(7943):249-250. doi: 10.1038/d41586-022-04449-y. Nature. 2023. PMID: 36544004 Free PMC article.
Similar articles
-
Caenorhabditis elegans BUB-3 and SAN-1/MAD3 Spindle Assembly Checkpoint Components Are Required for Genome Stability in Response to Treatment with Ionizing Radiation.G3 (Bethesda). 2017 Dec 4;7(12):3875-3885. doi: 10.1534/g3.117.1122. G3 (Bethesda). 2017. PMID: 29046436 Free PMC article.
-
DNA Strand Breaks in Mitotic Germ Cells of Caenorhabditis elegans Evaluated by Comet Assay.Mol Cells. 2016 Mar;39(3):204-10. doi: 10.14348/molcells.2016.2206. Epub 2016 Feb 23. Mol Cells. 2016. PMID: 26903030 Free PMC article.
-
Ionizing radiation and genetic risks XIV. Potential research directions in the post-genome era based on knowledge of repair of radiation-induced DNA double-strand breaks in mammalian somatic cells and the origin of deletions associated with human genomic disorders.Mutat Res. 2005 Oct 15;578(1-2):333-70. doi: 10.1016/j.mrfmmm.2005.06.020. Epub 2005 Aug 5. Mutat Res. 2005. PMID: 16084534 Review.
-
The C. elegans SET-2/SET1 histone H3 Lys4 (H3K4) methyltransferase preserves genome stability in the germline.DNA Repair (Amst). 2017 Sep;57:139-150. doi: 10.1016/j.dnarep.2017.07.007. Epub 2017 Jul 29. DNA Repair (Amst). 2017. PMID: 28779964
-
Is non-homologous end-joining really an inherently error-prone process?PLoS Genet. 2014 Jan;10(1):e1004086. doi: 10.1371/journal.pgen.1004086. Epub 2014 Jan 16. PLoS Genet. 2014. PMID: 24453986 Free PMC article. Review.
Cited by
-
Feasibility of a Percutaneous and Non-Fluoroscopic Procedure for Transcatheter Mitral Valve Edge-to-Edge Repair.Rev Cardiovasc Med. 2023 Dec 12;24(12):346. doi: 10.31083/j.rcm2412346. eCollection 2023 Dec. Rev Cardiovasc Med. 2023. PMID: 39077069 Free PMC article.
-
Involvement of H2A variants in DNA damage response of zygotes.Cell Death Discov. 2024 May 14;10(1):231. doi: 10.1038/s41420-024-01999-0. Cell Death Discov. 2024. PMID: 38744857 Free PMC article.
-
BRCA1/BRC-1 and SMC-5/6 regulate DNA repair pathway engagement during Caenorhabditis elegans meiosis.Elife. 2024 Aug 8;13:e80687. doi: 10.7554/eLife.80687. Elife. 2024. PMID: 39115289 Free PMC article.
-
Paternal high-fat diet altered SETD2 gene methylation in sperm of F0 and F1 mice.Genes Nutr. 2023 Aug 19;18(1):12. doi: 10.1186/s12263-023-00731-4. Genes Nutr. 2023. PMID: 37598138 Free PMC article.
-
The fetal origins of metabolic health: exploring the association between newborn biological age and metabolism hormones in childhood.BMC Med. 2024 Oct 8;22(1):429. doi: 10.1186/s12916-024-03629-z. BMC Med. 2024. PMID: 39379967 Free PMC article.
References
-
- UNSCEAR. Hereditary Effects of Radiation. 2001 Report to the General Assembly, with Scientific Annex (United Nations, 2001).
-
- Wright, S. The Roles of Mutation, Inbreeding, Crossbreeding, and Selection in Evolution. In: Proc. of the XI International Congress of Genetics8, 209–222 (1932).
Publication types
MeSH terms
Substances
LinkOut - more resources
Full Text Sources
Research Materials
Miscellaneous