The guanine nucleotide exchange factor Vav3 intervenes in the migration pathway of oligodendrocyte precursor cells on tenascin-C
- PMID: 36531963
- PMCID: PMC9748482
- DOI: 10.3389/fcell.2022.1042403
The guanine nucleotide exchange factor Vav3 intervenes in the migration pathway of oligodendrocyte precursor cells on tenascin-C
Abstract
Oligodendrocyte precursor cells (OPCs) are the exclusive source of myelination in the central nervous system (CNS). Prior to myelination, OPCs migrate to target areas and mature into myelinating oligodendrocytes. This process is underpinned by drastic changes of the cytoskeleton and partially driven by pathways involving small GTPases of the Rho subfamily. In general, the myelination process requires migration, proliferation and differentiation of OPCs. Presently, these processes are only partially understood. In this study, we analyzed the impact of the guanine nucleotide exchange factor (GEF) Vav3 on the migration behavior of OPCs. Vav3 is known to regulate RhoA, Rac1 and RhoG activity and is therefore a promising candidate with regard to a regulatory role concerning the rearrangement of the cytoskeleton. Our study focused on the Vav3 knockout mouse and revealed an enhanced migration capacity of Vav3 -/- OPCs on the extracellular matrix (ECM) glycoprotein tenascin-C (TnC). The migration behavior of individual OPCs on further ECM molecules such as laminin-1 (Ln1), laminin-2 (Ln2) and tenascin-R (TnR) was not affected by the elimination of Vav3. The migration process was further investigated with regard to intracellular signal transmission by pharmacological blockade of downstream pathways of specific Rho GTPases. Our data suggest that activation of RhoA GTPase signaling compromises migration, as inhibition of RhoA-signaling promoted migration behavior. This study provides novel insights into the control of OPC migration, which could be useful for further understanding of the complex differentiation and myelination process.
Keywords: Rho GTPase; Vav3; extracellular matrix; glia; laminin; migration; oligodendrocyte precursor cell; tenascin.
Copyright © 2022 Schäfer, Bauch, Wegrzyn, Roll, van Leeuwen, Jarocki and Faissner.
Conflict of interest statement
The authors declare that the research was conducted in the absence of any commercial or financial relationships that could be construed as a potential conflict of interest.
Figures
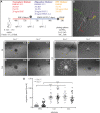
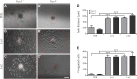
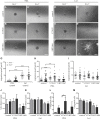
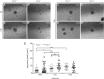
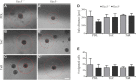
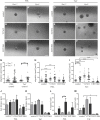
Similar articles
-
The guanine nucleotide exchange factor Vav3 modulates oligodendrocyte precursor differentiation and supports remyelination in white matter lesions.Glia. 2019 Feb;67(2):376-392. doi: 10.1002/glia.23548. Epub 2018 Nov 18. Glia. 2019. PMID: 30450647
-
The Extracellular Matrix Proteins Tenascin-C and Tenascin-R Retard Oligodendrocyte Precursor Maturation and Myelin Regeneration in a Cuprizone-Induced Long-Term Demyelination Animal Model.Cells. 2022 May 28;11(11):1773. doi: 10.3390/cells11111773. Cells. 2022. PMID: 35681468 Free PMC article.
-
NMDA receptor couples Rac1-GEF Tiam1 to direct oligodendrocyte precursor cell migration.Glia. 2013 Dec;61(12):2078-99. doi: 10.1002/glia.22578. Epub 2013 Oct 7. Glia. 2013. PMID: 24123220
-
Involvement of the guanine nucleotide exchange factor Vav3 in central nervous system development and plasticity.Biol Chem. 2017 May 1;398(5-6):663-675. doi: 10.1515/hsz-2016-0275. Biol Chem. 2017. PMID: 28214347 Review.
-
VAV3 in human cancers: Mechanism and clinical implication.Pathol Res Pract. 2023 Aug;248:154681. doi: 10.1016/j.prp.2023.154681. Epub 2023 Jul 13. Pathol Res Pract. 2023. PMID: 37467637 Review.
Cited by
-
Substrate-bound and soluble domains of tenascin-C regulate differentiation, proliferation and migration of neural stem and progenitor cells.Front Cell Neurosci. 2024 Feb 14;18:1357499. doi: 10.3389/fncel.2024.1357499. eCollection 2024. Front Cell Neurosci. 2024. PMID: 38425428 Free PMC article.
References
-
- Aoki K., Nakamura T., Fujikawa K., Matsuda M. (2005). Local phosphatidylinositol 3, 4, 5-trisphosphate accumulation recruits Vav2 and Vav3 to activate Rac1/Cdc42 and initiate neurite outgrowth in nerve growth factor-stimulated PC12 cells. Mol. Biol. Cell 16 (5), 2207–2217. 10.1091/mbc.E04-10-0904 - DOI - PMC - PubMed
-
- Bartsch U., Faissner A., Trotter J., Dörries U., Bartsch S., Mohajeri H., et al. (1994). Tenascin demarcates the boundary between the myelinated and nonmyelinated part of retinal ganglion cell axons in the developing and adult mouse. J. Neurosci. 14 (8), 4756–4768. 10.1523/jneurosci.14-08-04756.1994 - DOI - PMC - PubMed
LinkOut - more resources
Full Text Sources
Research Materials
Miscellaneous