Rapid induction and long-term self-renewal of neural crest-derived ectodermal chondrogenic cells from hPSCs
- PMID: 36477591
- PMCID: PMC9729200
- DOI: 10.1038/s41536-022-00265-0
Rapid induction and long-term self-renewal of neural crest-derived ectodermal chondrogenic cells from hPSCs
Abstract
Articular cartilage is highly specific and has limited capacity for regeneration if damaged. Human pluripotent stem cells (hPSCs) have the potential to generate any cell type in the body. Here, we report the dual-phase induction of ectodermal chondrogenic cells (ECCs) from hPSCs through the neural crest (NC). ECCs were able to self-renew long-term (over numerous passages) in a cocktail of growth factors and small molecules. The cells stably expressed cranial neural crest-derived mandibular condylar cartilage markers, such as MSX1, FOXC1 and FOXC2. Compared with chondroprogenitors from iPSCs via the paraxial mesoderm, ECCs had single-cell transcriptome profiles similar to condylar chondrocytes. After the removal of the cocktail sustaining self-renewal, the cells stopped proliferating and differentiated into a homogenous chondrocyte population. Remarkably, after transplantation, this cell lineage was able to form cartilage-like structures resembling mandibular condylar cartilage in vivo. This finding provides a framework to generate self-renewing cranial chondrogenic progenitors, which could be useful for developing cell-based therapy for cranial cartilage injury.
© 2022. The Author(s).
Conflict of interest statement
The authors declare no competing interests.
Figures
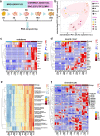
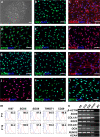
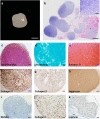
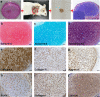
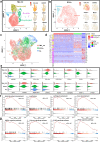
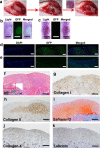
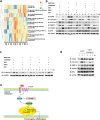
Similar articles
-
Human pluripotent stem cell-derived chondroprogenitors for cartilage tissue engineering.Cell Mol Life Sci. 2020 Jul;77(13):2543-2563. doi: 10.1007/s00018-019-03445-2. Epub 2020 Jan 8. Cell Mol Life Sci. 2020. PMID: 31915836 Free PMC article. Review.
-
Pre-transplantational Control of the Post-transplantational Fate of Human Pluripotent Stem Cell-Derived Cartilage.Stem Cell Reports. 2018 Aug 14;11(2):440-453. doi: 10.1016/j.stemcr.2018.06.021. Epub 2018 Jul 26. Stem Cell Reports. 2018. PMID: 30057264 Free PMC article.
-
Superficial cells are self-renewing chondrocyte progenitors, which form the articular cartilage in juvenile mice.FASEB J. 2017 Mar;31(3):1067-1084. doi: 10.1096/fj.201600918R. Epub 2016 Dec 13. FASEB J. 2017. PMID: 27965322 Free PMC article.
-
Cord blood cell-derived iPSCs as a new candidate for chondrogenic differentiation and cartilage regeneration.Stem Cell Res Ther. 2017 Jan 28;8(1):16. doi: 10.1186/s13287-017-0477-6. Stem Cell Res Ther. 2017. PMID: 28129782 Free PMC article.
-
The Potency of Induced Pluripotent Stem Cells in Cartilage Regeneration and Osteoarthritis Treatment.Adv Exp Med Biol. 2018;1079:55-68. doi: 10.1007/5584_2017_141. Adv Exp Med Biol. 2018. PMID: 29270885 Review.
Cited by
-
Craniofacial chondrogenesis in organoids from human stem cell-derived neural crest cells.iScience. 2024 Mar 28;27(4):109585. doi: 10.1016/j.isci.2024.109585. eCollection 2024 Apr 19. iScience. 2024. PMID: 38623327 Free PMC article.
-
Single-cell RNA sequencing reveals neurovascular-osteochondral network crosstalk during temporomandibular joint osteoarthritis: Pilot study in a human condylar cartilage.Heliyon. 2023 Oct 6;9(10):e20749. doi: 10.1016/j.heliyon.2023.e20749. eCollection 2023 Oct. Heliyon. 2023. PMID: 37867837 Free PMC article.
References
-
- Correa D, Lietman SA. Articular cartilage repair: current needs, methods and research directions. Semin. Cell Dev. Biol. 2017;62:67–77. - PubMed
-
- Barry F, Murphy M. Mesenchymal stem cells in joint disease and repair. Nat. Rev. Rheumatol. 2013;9:584–594. - PubMed
-
- Oldershaw RA, et al. Directed differentiation of human embryonic stem cells toward chondrocytes. Nat. Biotechnol. 2010;28:1187–1194. - PubMed
-
- Craft AM, et al. Generation of articular chondrocytes from human pluripotent stem cells. Nat. Biotechnol. 2015;33:638–645. - PubMed
LinkOut - more resources
Full Text Sources
Other Literature Sources
Research Materials