Derivation of nociceptive sensory neurons from hiPSCs with early patterning and temporally controlled NEUROG2 overexpression
- PMID: 36452863
- PMCID: PMC9701618
- DOI: 10.1016/j.crmeth.2022.100341
Derivation of nociceptive sensory neurons from hiPSCs with early patterning and temporally controlled NEUROG2 overexpression
Abstract
Despite development of protocols to differentiate human pluripotent stem cells (hPSCs), those used to produce sensory neurons remain difficult to replicate and result in heterogenous populations. There is a growing clinical burden of chronic pain conditions, highlighting the need for relevant human cellular models. This study presents a hybrid differentiation method to produce nociceptive sensory neurons from hPSCs. Lines harboring an inducible NEUROG2 construct were patterned toward precursors with small molecules followed by NEUROG2 overexpression. Neurons expressed key markers, including BRN3A and ISL1, with single-cell RNA sequencing, revealing populations of nociceptors expressing SCN9A and TRP channels. Physiological profiling with multi-electrode arrays revealed that neurons responded to noxious stimuli, including capsaicin. Finally, we modeled pain-like states to identify genes and pathways involved in pain transduction. This study presents an optimized method to efficiently produce nociceptive sensory neurons and provides a tool to aid development of chronic pain research.
Keywords: chronic pain models; electrophysiology; human iPSC; iPSC differentiation; multi-electrode array platform; sensory neurons; single-cell RNA-seq.
© 2022 The Author(s).
Conflict of interest statement
E.M. is an employee and shareholder of bit.bio.
Figures
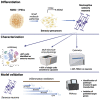
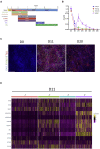
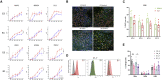
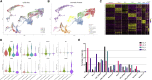
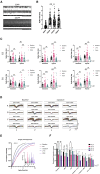
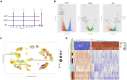
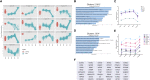
Similar articles
-
Satellite Glial Cells Give Rise to Nociceptive Sensory Neurons.Stem Cell Rev Rep. 2021 Jun;17(3):999-1013. doi: 10.1007/s12015-020-10102-w. Epub 2021 Jan 3. Stem Cell Rev Rep. 2021. PMID: 33389681
-
The role of Nav1.7 in human nociceptors: insights from human induced pluripotent stem cell-derived sensory neurons of erythromelalgia patients.Pain. 2019 Jun;160(6):1327-1341. doi: 10.1097/j.pain.0000000000001511. Pain. 2019. PMID: 30720580 Free PMC article.
-
Visceral and somatic pain modalities reveal NaV 1.7-independent visceral nociceptive pathways.J Physiol. 2017 Apr 15;595(8):2661-2679. doi: 10.1113/JP272837. Epub 2017 Mar 1. J Physiol. 2017. PMID: 28105664 Free PMC article.
-
The Role of Voltage-Gated Sodium Channels in Pain Signaling.Physiol Rev. 2019 Apr 1;99(2):1079-1151. doi: 10.1152/physrev.00052.2017. Physiol Rev. 2019. PMID: 30672368 Review.
-
Recent advances for using human induced-pluripotent stem cells as pain-in-a-dish models of neuropathic pain.Exp Neurol. 2022 Dec;358:114223. doi: 10.1016/j.expneurol.2022.114223. Epub 2022 Sep 12. Exp Neurol. 2022. PMID: 36100046 Review.
Cited by
-
The pain target NaV1.7 is expressed late during human iPS cell differentiation into sensory neurons as determined in high-resolution imaging.Pflugers Arch. 2024 Jun;476(6):975-992. doi: 10.1007/s00424-024-02945-w. Epub 2024 Mar 27. Pflugers Arch. 2024. PMID: 38538988 Free PMC article.
-
ISL1 and AQP5 complement each other to enhance gastric cancer cell stemness by regulating CD44 expression.Transl Cancer Res. 2024 Oct 31;13(10):5484-5496. doi: 10.21037/tcr-24-248. Epub 2024 Oct 28. Transl Cancer Res. 2024. PMID: 39525012 Free PMC article.
References
Publication types
MeSH terms
Substances
LinkOut - more resources
Full Text Sources
Medical
Molecular Biology Databases