Reconstitution of a Reversible Membrane Switch via Prenylation by One-Pot Cell-Free Expression
- PMID: 36445320
- PMCID: PMC9872162
- DOI: 10.1021/acssynbio.2c00406
Reconstitution of a Reversible Membrane Switch via Prenylation by One-Pot Cell-Free Expression
Abstract
Reversible membrane targeting of proteins is one of the key regulators of cellular interaction networks, for example, for signaling and polarization. So-called "membrane switches" are thus highly attractive targets for the design of minimal cells but have so far been tricky to reconstitute in vitro. Here, we introduce cell-free prenylated protein synthesis (CFpPS), which enables the synthesis and membrane targeting of proteins in a single reaction mix including the prenylation machinery. CFpPS can confer membrane affinity to any protein via addition of a 4-peptide motif to its C-terminus and offers robust production of prenylated proteins not only in their soluble forms but also in the direct vicinity of biomimetic membranes. Thus, CFpPS enabled us to reconstitute the prenylated polarity hub Cdc42 and its regulatory protein in vitro, implementing a key membrane switch. We propose CFpPS to be a versatile and effective platform for engineering complex features, such as polarity induction, in synthetic cells.
Keywords: Cdc42; cell-free protein synthesis; prenylation; reversible membrane switch; synthetic biology; synthetic cell.
Conflict of interest statement
The authors declare no competing financial interest.
Figures
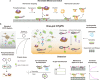
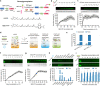
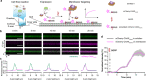
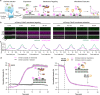
Similar articles
-
Multifunctional prenylated peptides for live cell analysis.J Am Chem Soc. 2009 Jun 3;131(21):7293-303. doi: 10.1021/ja805174z. J Am Chem Soc. 2009. PMID: 19425596 Free PMC article.
-
Prenylation and membrane localization of Cdc42 are essential for activation by DOCK7.Biochemistry. 2013 Jun 25;52(25):4354-63. doi: 10.1021/bi301688g. Epub 2013 Jun 14. Biochemistry. 2013. PMID: 23718289 Free PMC article.
-
Microsomal membranes contain a high affinity binding site for prenylated peptides.J Biol Chem. 1993 Jul 5;268(19):13780-3. J Biol Chem. 1993. PMID: 8314746
-
Chemical biology of protein lipidation: semi-synthesis and structure elucidation of prenylated RabGTPases.Org Biomol Chem. 2005 Apr 7;3(7):1157-64. doi: 10.1039/b417573e. Epub 2005 Feb 24. Org Biomol Chem. 2005. PMID: 15785799 Review.
-
Updates on protein-prenylation and associated inherited retinopathies.Front Ophthalmol (Lausanne). 2024 Jul 4;4:1410874. doi: 10.3389/fopht.2024.1410874. eCollection 2024. Front Ophthalmol (Lausanne). 2024. PMID: 39026984 Free PMC article. Review.
Cited by
-
Advancing synthetic biology through cell-free protein synthesis.Comput Struct Biotechnol J. 2023 May 4;21:2899-2908. doi: 10.1016/j.csbj.2023.05.003. eCollection 2023. Comput Struct Biotechnol J. 2023. PMID: 37216017 Free PMC article. Review.
-
Bottom-Up Synthetic Biology Using Cell-Free Protein Synthesis.Adv Biochem Eng Biotechnol. 2023;185:1-20. doi: 10.1007/10_2023_232. Adv Biochem Eng Biotechnol. 2023. PMID: 37526707 Review.
-
Lipophilic Anchors that Embed Bioconjugates in Bilayer Membranes: A Review.Bioconjug Chem. 2023 Jun 21;34(6):961-971. doi: 10.1021/acs.bioconjchem.3c00204. Epub 2023 Jun 5. Bioconjug Chem. 2023. PMID: 37276240 Free PMC article. Review.
References
Publication types
MeSH terms
Substances
LinkOut - more resources
Full Text Sources
Miscellaneous