Electrically stimulated droplet injector for reduced sample consumption in serial crystallography
- PMID: 36425668
- PMCID: PMC9680787
- DOI: 10.1016/j.bpr.2022.100081
Electrically stimulated droplet injector for reduced sample consumption in serial crystallography
Abstract
With advances in X-ray free-electron lasers (XFELs), serial femtosecond crystallography (SFX) has enabled the static and dynamic structure determination for challenging proteins such as membrane protein complexes. In SFX with XFELs, the crystals are typically destroyed after interacting with a single XFEL pulse. Therefore, thousands of new crystals must be sequentially introduced into the X-ray beam to collect full data sets. Because of the serial nature of any SFX experiment, up to 99% of the sample delivered to the X-ray beam during its "off-time" between X-ray pulses is wasted due to the intrinsic pulsed nature of all current XFELs. To solve this major problem of large and often limiting sample consumption, we report on improvements of a revolutionary sample-saving method that is compatible with all current XFELs. We previously reported 3D-printed injection devices coupled with gas dynamic virtual nozzles (GDVNs) capable of generating samples containing droplets segmented by an immiscible oil phase for jetting crystal-laden droplets into the path of an XFEL. Here, we have further improved the device design by including metal electrodes inducing electrowetting effects for improved control over droplet generation frequency to stimulate the droplet release to matching the XFEL repetition rate by employing an electrical feedback mechanism. We report the improvements in this electrically triggered segmented flow approach for sample conservation in comparison with a continuous GDVN injection using the microcrystals of lysozyme and 3-deoxy-D-manno-octulosonate 8-phosphate synthase and report the segmented flow approach for sample injection applied at the Macromolecular Femtosecond Crystallography instrument at the Linear Coherent Light Source for the first time.
© 2022 The Authors.
Conflict of interest statement
A.E.G., J.C.V., and A.R. hold a patent on electrical droplet stimulation in a 3D-printed device.
Figures
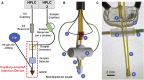
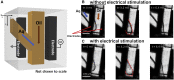
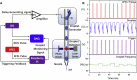
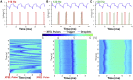
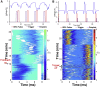
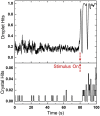
Similar articles
-
Segmented flow generator for serial crystallography at the European X-ray free electron laser.Nat Commun. 2020 Sep 9;11(1):4511. doi: 10.1038/s41467-020-18156-7. Nat Commun. 2020. PMID: 32908128 Free PMC article.
-
3D printed droplet generation devices for serial femtosecond crystallography enabled by surface coating.J Appl Crystallogr. 2019 Aug 29;52(Pt 5):997-1008. doi: 10.1107/S1600576719010343. eCollection 2019 Oct 1. J Appl Crystallogr. 2019. PMID: 31636518 Free PMC article.
-
Electric Triggering for Enhanced Control of Droplet Generation.Anal Chem. 2019 Aug 6;91(15):9792-9799. doi: 10.1021/acs.analchem.9b01449. Epub 2019 Jul 16. Anal Chem. 2019. PMID: 31260621 Free PMC article.
-
Femtosecond crystallography of membrane proteins in the lipidic cubic phase.Philos Trans R Soc Lond B Biol Sci. 2014 Jul 17;369(1647):20130314. doi: 10.1098/rstb.2013.0314. Philos Trans R Soc Lond B Biol Sci. 2014. PMID: 24914147 Free PMC article. Review.
-
Dynamic Structural Biology Experiments at XFEL or Synchrotron Sources.Methods Mol Biol. 2021;2305:203-228. doi: 10.1007/978-1-0716-1406-8_11. Methods Mol Biol. 2021. PMID: 33950392 Review.
Cited by
-
Bridging the microscopic divide: a comprehensive overview of micro-crystallization and in vivo crystallography.IUCrJ. 2024 Jul 1;11(Pt 4):476-485. doi: 10.1107/S205225252400513X. IUCrJ. 2024. PMID: 38958014 Free PMC article. Review.
-
Advanced manufacturing provides tailor-made solutions for crystallography with x-ray free-electron lasers.Struct Dyn. 2024 Feb 21;11(1):011101. doi: 10.1063/4.0000229. eCollection 2024 Jan. Struct Dyn. 2024. PMID: 38389979 Free PMC article.
-
Droplet microfluidics for time-resolved serial crystallography.IUCrJ. 2024 Mar 1;11(Pt 2):237-248. doi: 10.1107/S2052252524001799. IUCrJ. 2024. PMID: 38446456 Free PMC article.
-
Review of serial femtosecond crystallography including the COVID-19 pandemic impact and future outlook.Structure. 2023 Nov 2;31(11):1306-1319. doi: 10.1016/j.str.2023.10.005. Epub 2023 Oct 27. Structure. 2023. PMID: 37898125 Free PMC article. Review.
-
Room-temperature structural studies of SARS-CoV-2 protein NendoU with an X-ray free-electron laser.Structure. 2023 Feb 2;31(2):138-151.e5. doi: 10.1016/j.str.2022.12.009. Epub 2023 Jan 10. Structure. 2023. PMID: 36630960 Free PMC article.
References
-
- Lee J.-H., Zatsepin N.A., Kim K.H. Time-resolved serial femtosecond X-ray crystallography. BioDesign. 2018;6:15–22.
Grants and funding
LinkOut - more resources
Full Text Sources