The Obligate Intracellular Bacterial Pathogen Anaplasma phagocytophilum Exploits Host Cell Multivesicular Body Biogenesis for Proliferation and Dissemination
- PMID: 36409075
- PMCID: PMC9765717
- DOI: 10.1128/mbio.02961-22
The Obligate Intracellular Bacterial Pathogen Anaplasma phagocytophilum Exploits Host Cell Multivesicular Body Biogenesis for Proliferation and Dissemination
Abstract
Anaplasma phagocytophilum is the etiologic agent of the emerging infection, granulocytic anaplasmosis. This obligate intracellular bacterium lives in a host cell-derived vacuole that receives membrane traffic from multiple organelles to fuel its proliferation and from which it must ultimately exit to disseminate infection. Understanding of these essential pathogenic mechanisms has remained poor. Multivesicular bodies (MVBs) are late endosomal compartments that receive biomolecules from other organelles and encapsulate them into intralumenal vesicles (ILVs) using endosomal sorting complexes required for transport (ESCRT) machinery and ESCRT-independent machinery. Association of the ESCRT-independent protein, ALIX, directs MVBs to the plasma membrane where they release ILVs as exosomes. We report that the A. phagocytophilum vacuole (ApV) is acidified and enriched in lysobisphosphatidic acid, a lipid that is abundant in MVBs. ESCRT-0 and ESCRT-III components along with ALIX localize to the ApV membrane. siRNA-mediated inactivation of ESCRT-0 and ALIX together impairs A. phagocytophilum proliferation and infectious progeny production. RNA silencing of ESCRT-III, which regulates ILV scission, pronouncedly reduces ILV formation in ApVs and halts infection by arresting bacterial growth. Rab27a and its effector Munc13-4, which drive MVB trafficking to the plasma membrane and subsequent exosome release, localize to the ApV. Treatment with Nexinhib20, a small molecule inhibitor that specifically targets Rab27a to block MVB exocytosis, abrogates A. phagocytophilum infectious progeny release. Thus, A. phagocytophilum exploits MVB biogenesis and exosome release to benefit each major stage of its intracellular infection cycle: intravacuolar growth, conversion to the infectious form, and exit from the host cell. IMPORTANCE Anaplasma phagocytophilum causes granulocytic anaplasmosis, a globally emerging zoonosis that can be severe, even fatal, and for which antibiotic treatment options are limited. A. phagocytophilum lives in an endosomal-like compartment that interfaces with multiple organelles and from which it must ultimately exit to spread within the host. How the bacterium accomplishes these tasks is poorly understood. Multivesicular bodies (MVBs) are intermediates in the endolysosomal pathway that package biomolecular cargo from other organelles as intralumenal vesicles for release at the plasma membrane as exosomes. We discovered that A. phagocytophilum exploits MVB biogenesis and trafficking to benefit all aspects of its intracellular infection cycle: proliferation, conversion to its infectious form, and release of infectious progeny. The ability of a small molecule inhibitor of MVB exocytosis to impede A. phagocytophilum dissemination indicates the potential of this pathway as a novel host-directed therapeutic target for granulocytic anaplasmosis.
Keywords: Anaplasma phagocytophilum; ESCRT; Munc13-4; Rab27a; exocytosis; exosome; intralumenal vesicle; multivesicular body; multivesicular endosome; nutritional virulence; obligate intracellular bacterium; rickettsia; vacuolar pathogen.
Conflict of interest statement
The authors declare no conflict of interest.
Figures
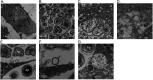
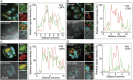
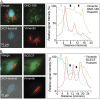
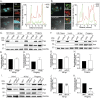
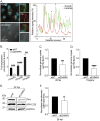
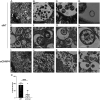
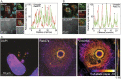
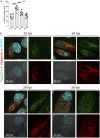
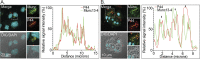
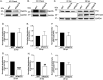
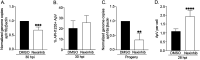
Similar articles
-
Bro1 stimulates Vps4 to promote intralumenal vesicle formation during multivesicular body biogenesis.J Cell Biol. 2021 Aug 2;220(8):e202102070. doi: 10.1083/jcb.202102070. Epub 2021 Jun 23. J Cell Biol. 2021. PMID: 34160559 Free PMC article.
-
The Pathogen-Occupied Vacuoles of Anaplasma phagocytophilum and Anaplasma marginale Interact with the Endoplasmic Reticulum.Front Cell Infect Microbiol. 2016 Mar 1;6:22. doi: 10.3389/fcimb.2016.00022. eCollection 2016. Front Cell Infect Microbiol. 2016. PMID: 26973816 Free PMC article.
-
Rab27a via its effector JFC1 localizes to Anaplasma inclusions and promotes Anaplasma proliferation in leukocytes.Microbes Infect. 2025 Jan;27(1):105278. doi: 10.1016/j.micinf.2023.105278. Epub 2023 Dec 16. Microbes Infect. 2025. PMID: 38110148
-
ALIX and the multivesicular endosome: ALIX in Wonderland.Trends Cell Biol. 2014 Jan;24(1):19-25. doi: 10.1016/j.tcb.2013.10.009. Epub 2013 Nov 26. Trends Cell Biol. 2014. PMID: 24287454 Review.
-
MVB vesicle formation: ESCRT-dependent, ESCRT-independent and everything in between.Curr Opin Cell Biol. 2011 Aug;23(4):452-7. doi: 10.1016/j.ceb.2011.04.008. Epub 2011 May 11. Curr Opin Cell Biol. 2011. PMID: 21570275 Free PMC article. Review.
Cited by
-
Innate immunity in rickettsial infections.Front Cell Infect Microbiol. 2023 May 9;13:1187267. doi: 10.3389/fcimb.2023.1187267. eCollection 2023. Front Cell Infect Microbiol. 2023. PMID: 37228668 Free PMC article. Review.
-
Ceramide-1-phosphate is a regulator of Golgi structure and is co-opted by the obligate intracellular bacterial pathogen Anaplasma phagocytophilum.mBio. 2024 Apr 10;15(4):e0029924. doi: 10.1128/mbio.00299-24. Epub 2024 Feb 28. mBio. 2024. PMID: 38415594 Free PMC article.
-
Tick extracellular vesicles in host skin immunity and pathogen transmission.Trends Parasitol. 2023 Oct;39(10):873-885. doi: 10.1016/j.pt.2023.07.009. Epub 2023 Aug 16. Trends Parasitol. 2023. PMID: 37591719 Free PMC article. Review.
-
The multifunction Coxiella effector Vice stimulates macropinocytosis and interferes with the ESCRT machinery.Proc Natl Acad Sci U S A. 2024 Jun 18;121(25):e2315481121. doi: 10.1073/pnas.2315481121. Epub 2024 Jun 13. Proc Natl Acad Sci U S A. 2024. PMID: 38870060 Free PMC article.
-
Rab27 in tick extracellular vesicle biogenesis and infection.bioRxiv [Preprint]. 2023 Nov 2:2023.11.02.565357. doi: 10.1101/2023.11.02.565357. bioRxiv. 2023. Update in: Parasit Vectors. 2024 Feb 9;17(1):57. doi: 10.1186/s13071-024-06150-7. PMID: 37961338 Free PMC article. Updated. Preprint.
References
MeSH terms
Substances
Grants and funding
LinkOut - more resources
Full Text Sources
Miscellaneous