The mechanical cell - the role of force dependencies in synchronising protein interaction networks
- PMID: 36398718
- PMCID: PMC9845749
- DOI: 10.1242/jcs.259769
The mechanical cell - the role of force dependencies in synchronising protein interaction networks
Abstract
The role of mechanical signals in the proper functioning of organisms is increasingly recognised, and every cell senses physical forces and responds to them. These forces are generated both from outside the cell or via the sophisticated force-generation machinery of the cell, the cytoskeleton. All regions of the cell are connected via mechanical linkages, enabling the whole cell to function as a mechanical system. In this Review, we define some of the key concepts of how this machinery functions, highlighting the critical requirement for mechanosensory proteins, and conceptualise the coupling of mechanical linkages to mechanochemical switches that enables forces to be converted into biological signals. These mechanical couplings provide a mechanism for how mechanical crosstalk might coordinate the entire cell, its neighbours, extending into whole collections of cells, in tissues and in organs, and ultimately in the coordination and operation of entire organisms. Consequently, many diseases manifest through defects in this machinery, which we map onto schematics of the mechanical linkages within a cell. This mapping approach paves the way for the identification of additional linkages between mechanosignalling pathways and so might identify treatments for diseases, where mechanical connections are affected by mutations or where individual force-regulated components are defective.
Keywords: Cytoskeleton; Disease-associated mutations; Force-dependent switches; Mechanosensing; Mechanotransduction; Motor proteins; Talin.
© 2022. Published by The Company of Biologists Ltd.
Conflict of interest statement
Competing interests The authors declare no competing or financial interests.
Figures
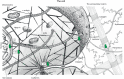
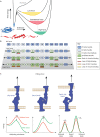
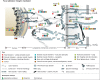
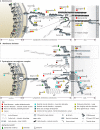
Similar articles
-
Nuclear forces and cell mechanosensing.Prog Mol Biol Transl Sci. 2014;126:205-15. doi: 10.1016/B978-0-12-394624-9.00008-7. Prog Mol Biol Transl Sci. 2014. PMID: 25081619 Free PMC article. Review.
-
Mechanosensing and mechanochemical transduction: how is mechanical energy sensed and converted into chemical energy in an extracellular matrix?Crit Rev Biomed Eng. 2003;31(4):255-331. doi: 10.1615/critrevbiomedeng.v31.i4.10. Crit Rev Biomed Eng. 2003. PMID: 15095950 Review.
-
The matrix environmental and cell mechanical properties regulate cell migration and contribute to the invasive phenotype of cancer cells.Rep Prog Phys. 2019 Jun;82(6):064602. doi: 10.1088/1361-6633/ab1628. Epub 2019 Apr 4. Rep Prog Phys. 2019. PMID: 30947151 Review.
-
Molecular mechanism for direct actin force-sensing by α-catenin.Elife. 2020 Sep 24;9:e62514. doi: 10.7554/eLife.62514. Elife. 2020. PMID: 32969337 Free PMC article.
-
Mechanical regulation of a molecular clutch defines force transmission and transduction in response to matrix rigidity.Nat Cell Biol. 2016 May;18(5):540-8. doi: 10.1038/ncb3336. Epub 2016 Apr 11. Nat Cell Biol. 2016. PMID: 27065098
Cited by
-
Force-dependent focal adhesion assembly and disassembly: A computational study.PLoS Comput Biol. 2023 Oct 6;19(10):e1011500. doi: 10.1371/journal.pcbi.1011500. eCollection 2023 Oct. PLoS Comput Biol. 2023. PMID: 37801464 Free PMC article.
-
Mechanically operated signalling scaffolds.Biochem Soc Trans. 2024 Apr 24;52(2):517-527. doi: 10.1042/BST20221194. Biochem Soc Trans. 2024. PMID: 38572868 Free PMC article. Review.
-
Molecular dynamics simulations reveal how vinculin refolds partially unfolded talin rod helices to stabilize them against mechanical force.PLoS Comput Biol. 2024 Aug 7;20(8):e1012341. doi: 10.1371/journal.pcbi.1012341. eCollection 2024 Aug. PLoS Comput Biol. 2024. PMID: 39110765 Free PMC article.
-
The focal adhesion protein talin is a mechanically gated A-kinase anchoring protein.Proc Natl Acad Sci U S A. 2024 Mar 26;121(13):e2314947121. doi: 10.1073/pnas.2314947121. Epub 2024 Mar 21. Proc Natl Acad Sci U S A. 2024. PMID: 38513099 Free PMC article.
-
Laminin-α2 chain deficiency in skeletal muscle causes dysregulation of multiple cellular mechanisms.Life Sci Alliance. 2024 Oct 8;7(12):e202402829. doi: 10.26508/lsa.202402829. Print 2024 Dec. Life Sci Alliance. 2024. PMID: 39379105 Free PMC article.
References
-
- Acsadi, G., Moore, S. A., Chéron, A., Delalande, O., Bennett, L., Kupsky, W., El-Baba, M., Le Rumeur, E. and Hubert, J. F. (2012). Novel mutation in spectrin-like repeat 1 of dystrophin central domain causes protein misfolding and mild Becker muscular dystrophy. J. Biol. Chem. 287, 18153-18162. 10.1074/jbc.M111.284521 - DOI - PMC - PubMed
Publication types
MeSH terms
Grants and funding
LinkOut - more resources
Full Text Sources