Cross-reactive neutralizing human monoclonal antibodies mapping to variable antigenic sites on the norovirus major capsid protein
- PMID: 36389818
- PMCID: PMC9641292
- DOI: 10.3389/fimmu.2022.1040836
Cross-reactive neutralizing human monoclonal antibodies mapping to variable antigenic sites on the norovirus major capsid protein
Abstract
Human noroviruses are the major viral cause of acute gastroenteritis around the world. Although norovirus symptoms are in most cases mild and self-limited, severe and prolonged symptoms can occur in the elderly and in immunocompromised individuals. Thus, there is a great need for the development of specific therapeutics that can help mitigate infection. In this study, we sought to characterize a panel of human monoclonal antibodies (mAbs; NORO-123, -115, -273A, -263, -315B, and -250B) that showed carbohydrate blocking activity against the current pandemic variant, GII.4 Sydney 2012. All antibodies tested showed potent neutralization against GII.4 Sydney virus in human intestinal enteroid culture. While all mAbs recognized only GII.4 viruses, they exhibited differential binding patterns against a panel of virus-like particles (VLPs) representing major and minor GII.4 variants spanning twenty-five years. Using mutant VLPs, we mapped five of the mAbs to variable antigenic sites A (NORO-123, -263, -315B, and -250B) or C (NORO-115) on the major capsid protein. Those mapping to the antigenic site A showed blocking activity against multiple variants dating back to 1987, with one mAb (NORO-123) showing reactivity to all variants tested. NORO-115, which maps to antigenic site C, showed reactivity against multiple variants due to the low susceptibility for mutations presented by naturally-occurring variants at the proposed binding site. Notably, we show that cross-blocking and neutralizing antibodies can be elicited against variable antigenic sites. These data provide new insights into norovirus immunity and suggest potential for the development of cross-protective vaccines and therapeutics.
Keywords: GII.4; antibodies; cross-reactive; gastroenteritis; mapping; neutralization; norovirus.
Copyright © 2022 Ford-Siltz, Tohma, Alvarado, Kendra, Pilewski, Crowe and Parra.
Conflict of interest statement
JC has served as a consultant for Luna Labs USA, Merck Sharp & Dohme Corporation, Emergent Biosolutions, and GlaxoSmithKline, and is a member of the Scientific Advisory Board of Meissa Vaccines, a former member of the Scientific Advisory Board of Gigagen Grifols and is founder of IDBiologics. The laboratory of JC received unrelated sponsored research agreements from AstraZeneca, Takeda, and IDBiologics during the conduct of the study. Vanderbilt University has applied for patents for some of the antibodies in this paper. The remaining authors declare that the research was conducted in the absence of any commercial or financial relationships that could be construed as a potential conflict of interest.
Figures
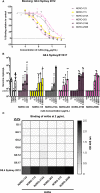
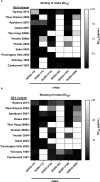
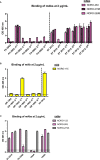
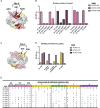
Similar articles
-
Minimal Antigenic Evolution after a Decade of Norovirus GII.4 Sydney_2012 Circulation in Humans.J Virol. 2023 Feb 28;97(2):e0171622. doi: 10.1128/jvi.01716-22. Epub 2023 Jan 23. J Virol. 2023. PMID: 36688654 Free PMC article.
-
Human Norovirus Neutralized by a Monoclonal Antibody Targeting the Histo-Blood Group Antigen Pocket.J Virol. 2019 Feb 19;93(5):e02174-18. doi: 10.1128/JVI.02174-18. Print 2019 Mar 1. J Virol. 2019. PMID: 30541855 Free PMC article.
-
Broadly cross-reactive human antibodies that inhibit genogroup I and II noroviruses.Nat Commun. 2021 Jul 14;12(1):4320. doi: 10.1038/s41467-021-24649-w. Nat Commun. 2021. PMID: 34262046 Free PMC article.
-
GII.4 Human Norovirus: Surveying the Antigenic Landscape.Viruses. 2019 Feb 20;11(2):177. doi: 10.3390/v11020177. Viruses. 2019. PMID: 30791623 Free PMC article. Review.
-
The Antigenic Topology of Norovirus as Defined by B and T Cell Epitope Mapping: Implications for Universal Vaccines and Therapeutics.Viruses. 2019 May 10;11(5):432. doi: 10.3390/v11050432. Viruses. 2019. PMID: 31083353 Free PMC article. Review.
Cited by
-
HuNoV Non-Structural Protein P22 Induces Maturation of IL-1β and IL-18 and N-GSDMD-Dependent Pyroptosis through Activating NLRP3 Inflammasome.Vaccines (Basel). 2023 May 17;11(5):993. doi: 10.3390/vaccines11050993. Vaccines (Basel). 2023. PMID: 37243097 Free PMC article.
-
Structural analyses of the GI.4 norovirus by cryo-electron microscopy and X-ray crystallography revealing binding sites for human monoclonal antibodies.J Virol. 2024 May 14;98(5):e0019724. doi: 10.1128/jvi.00197-24. Epub 2024 Apr 9. J Virol. 2024. PMID: 38593321 Free PMC article.
-
Human norovirus cultivation systems and their use in antiviral research.J Virol. 2024 Apr 16;98(4):e0166323. doi: 10.1128/jvi.01663-23. Epub 2024 Mar 12. J Virol. 2024. PMID: 38470106 Free PMC article. Review.
-
Insights into human norovirus cultivation in human intestinal enteroids.mSphere. 2024 Nov 21;9(11):e0044824. doi: 10.1128/msphere.00448-24. Epub 2024 Oct 15. mSphere. 2024. PMID: 39404443 Free PMC article.
References
Publication types
MeSH terms
Substances
Grants and funding
LinkOut - more resources
Full Text Sources
Medical