Multiple waves of viral invasions in Symbiodiniaceae algal genomes
- PMID: 36381231
- PMCID: PMC9651163
- DOI: 10.1093/ve/veac101
Multiple waves of viral invasions in Symbiodiniaceae algal genomes
Abstract
Dinoflagellates from the family Symbiodiniaceae are phototrophic marine protists that engage in symbiosis with diverse hosts. Their large and distinct genomes are characterized by pervasive gene duplication and large-scale retroposition events. However, little is known about the role and scale of horizontal gene transfer (HGT) in the evolution of this algal family. In other dinoflagellates, high levels of HGTs have been observed, linked to major genomic transitions, such as the appearance of a viral-acquired nucleoprotein that originated via HGT from a large DNA algal virus. Previous work showed that Symbiodiniaceae from different hosts are actively infected by viral groups, such as giant DNA viruses and ssRNA viruses, that may play an important role in coral health. Latent viral infections may also occur, whereby viruses could persist in the cytoplasm or integrate into the host genome as a provirus. This hypothesis received experimental support; however, the cellular localization of putative latent viruses and their taxonomic affiliation are still unknown. In addition, despite the finding of viral sequences in some genomes of Symbiodiniaceae, viral origin, taxonomic breadth, and metabolic potential have not been explored. To address these questions, we searched for putative viral-derived proteins in thirteen Symbiodiniaceae genomes. We found fifty-nine candidate viral-derived HGTs that gave rise to twelve phylogenies across ten genomes. We also describe the taxonomic affiliation of these virus-related sequences, their structure, and their genomic context. These results lead us to propose a model to explain the origin and fate of Symbiodiniaceae viral acquisitions.
Keywords: Symbiodiniaceae evolution; Symbiodinium virus; algal virus; dinoflagellate virus; virus horizontal gene transfer.
© The Author(s) 2022. Published by Oxford University Press.
Figures
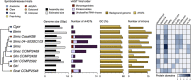
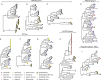
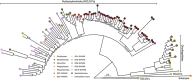
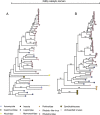
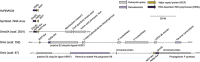
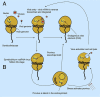
Similar articles
-
Gene clusters for biosynthesis of mycosporine-like amino acids in dinoflagellate nuclear genomes: Possible recent horizontal gene transfer between species of Symbiodiniaceae (Dinophyceae).J Phycol. 2022 Feb;58(1):1-11. doi: 10.1111/jpy.13219. Epub 2021 Nov 26. J Phycol. 2022. PMID: 34699617 Free PMC article. Review.
-
Comparison of 15 dinoflagellate genomes reveals extensive sequence and structural divergence in family Symbiodiniaceae and genus Symbiodinium.BMC Biol. 2021 Apr 13;19(1):73. doi: 10.1186/s12915-021-00994-6. BMC Biol. 2021. PMID: 33849527 Free PMC article.
-
Gene duplication is the primary driver of intraspecific genomic divergence in coral algal symbionts.Open Biol. 2023 Sep;13(9):230182. doi: 10.1098/rsob.230182. Epub 2023 Sep 27. Open Biol. 2023. PMID: 37751888 Free PMC article.
-
SAGER: a database of Symbiodiniaceae and Algal Genomic Resource.Database (Oxford). 2020 Jan 1;2020:baaa051. doi: 10.1093/database/baaa051. Database (Oxford). 2020. PMID: 32621601 Free PMC article.
-
Genome Evolution of Coral Reef Symbionts as Intracellular Residents.Trends Ecol Evol. 2019 Sep;34(9):799-806. doi: 10.1016/j.tree.2019.04.010. Epub 2019 May 10. Trends Ecol Evol. 2019. PMID: 31084944 Review.
Cited by
-
Predicted Membrane-Associated Domains in Proteins Encoded by Novel Monopartite Plant RNA Viruses Related to Members of the Family Benyviridae.Int J Mol Sci. 2023 Jul 29;24(15):12161. doi: 10.3390/ijms241512161. Int J Mol Sci. 2023. PMID: 37569537 Free PMC article.
-
In Situ Molecular Ecological Analyses Illuminate Distinct Factors Regulating Formation and Demise of a Harmful Dinoflagellate Bloom.Microbiol Spectr. 2023 Jun 15;11(3):e0515722. doi: 10.1128/spectrum.05157-22. Epub 2023 Apr 19. Microbiol Spectr. 2023. PMID: 37074171 Free PMC article.
References
LinkOut - more resources
Full Text Sources