Functional and molecular dissection of HCMV long non-coding RNAs
- PMID: 36369338
- PMCID: PMC9652368
- DOI: 10.1038/s41598-022-23317-3
Functional and molecular dissection of HCMV long non-coding RNAs
Abstract
Small, compact genomes confer a selective advantage to viruses, yet human cytomegalovirus (HCMV) expresses the long non-coding RNAs (lncRNAs); RNA1.2, RNA2.7, RNA4.9, and RNA5.0. Little is known about the function of these lncRNAs in the virus life cycle. Here, we dissected the functional and molecular landscape of HCMV lncRNAs. We found that HCMV lncRNAs occupy ~ 30% and 50-60% of total and poly(A)+viral transcriptome, respectively, throughout virus life cycle. RNA1.2, RNA2.7, and RNA4.9, the three abundantly expressed lncRNAs, appear to be essential in all infection states. Among these three lncRNAs, depletion of RNA2.7 and RNA4.9 results in the greatest defect in maintaining latent reservoir and promoting lytic replication, respectively. Moreover, we delineated the global post-transcriptional nature of HCMV lncRNAs by nanopore direct RNA sequencing and interactome analysis. We revealed that the lncRNAs are modified with N6-methyladenosine (m6A) and interact with m6A readers in all infection states. In-depth analysis demonstrated that m6A machineries stabilize HCMV lncRNAs, which could account for the overwhelming abundance of viral lncRNAs. Our study lays the groundwork for understanding the viral lncRNA-mediated regulation of host-virus interaction throughout the HCMV life cycle.
© 2022. The Author(s).
Conflict of interest statement
The authors declare no competing interests.
Figures
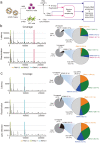
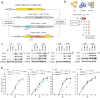
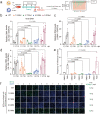
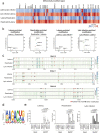
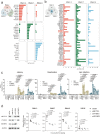
Similar articles
-
Insights into the Transcriptome of Human Cytomegalovirus: A Comprehensive Review.Viruses. 2023 Aug 8;15(8):1703. doi: 10.3390/v15081703. Viruses. 2023. PMID: 37632045 Free PMC article. Review.
-
Human cytomegalovirus long noncoding RNA4.9 regulates viral DNA replication.PLoS Pathog. 2020 Apr 15;16(4):e1008390. doi: 10.1371/journal.ppat.1008390. eCollection 2020 Apr. PLoS Pathog. 2020. PMID: 32294138 Free PMC article.
-
Human Cytomegalovirus RNA2.7 Is Required for Upregulating Multiple Cellular Genes To Promote Cell Motility and Viral Spread Late in Lytic Infection.J Virol. 2021 Sep 27;95(20):e0069821. doi: 10.1128/JVI.00698-21. Epub 2021 Aug 4. J Virol. 2021. PMID: 34346763 Free PMC article.
-
Transcriptome altered by latent human cytomegalovirus infection on THP-1 cells using RNA-seq.Gene. 2016 Dec 5;594(1):144-150. doi: 10.1016/j.gene.2016.09.014. Epub 2016 Sep 10. Gene. 2016. PMID: 27623506 Free PMC article.
-
MicroRNAs expressed by human cytomegalovirus.Virol J. 2020 Mar 12;17(1):34. doi: 10.1186/s12985-020-1296-4. Virol J. 2020. PMID: 32164742 Free PMC article. Review.
Cited by
-
Current Knowledge on the Interaction of Human Cytomegalovirus Infection, Encoded miRNAs, and Acute Aortic Syndrome.Viruses. 2023 Sep 29;15(10):2027. doi: 10.3390/v15102027. Viruses. 2023. PMID: 37896804 Free PMC article. Review.
-
Insights into the Transcriptome of Human Cytomegalovirus: A Comprehensive Review.Viruses. 2023 Aug 8;15(8):1703. doi: 10.3390/v15081703. Viruses. 2023. PMID: 37632045 Free PMC article. Review.
-
The hidden RNA code: implications of the RNA epitranscriptome in the context of viral infections.Front Genet. 2023 Aug 1;14:1245683. doi: 10.3389/fgene.2023.1245683. eCollection 2023. Front Genet. 2023. PMID: 37614818 Free PMC article. Review.
-
Chromatin control of human cytomegalovirus infection.mBio. 2023 Aug 31;14(4):e0032623. doi: 10.1128/mbio.00326-23. Epub 2023 Jul 13. mBio. 2023. PMID: 37439556 Free PMC article. Review.
-
Aid or Antagonize: Nuclear Long Noncoding RNAs Regulate Host Responses and Outcomes of Viral Infections.Cells. 2023 Mar 23;12(7):987. doi: 10.3390/cells12070987. Cells. 2023. PMID: 37048060 Free PMC article. Review.