Opaganib Protects against Radiation Toxicity: Implications for Homeland Security and Antitumor Radiotherapy
- PMID: 36361977
- PMCID: PMC9655569
- DOI: 10.3390/ijms232113191
Opaganib Protects against Radiation Toxicity: Implications for Homeland Security and Antitumor Radiotherapy
Abstract
Exposure to ionizing radiation (IR) is a lingering threat from accidental or terroristic nuclear events, but is also widely used in cancer therapy. In both cases, host inflammatory responses to IR damage normal tissue causing morbidity and possibly mortality to the victim/patient. Opaganib, a first-in-class inhibitor of sphingolipid metabolism, has broad anti-inflammatory and anticancer activity. Opaganib elevates ceramide and reduces sphingosine 1-phosphate (S1P) in cells, conditions that increase the antitumor efficacy of radiation while concomitantly suppressing inflammatory damage to normal tissue. Therefore, opaganib may suppress toxicity from unintended IR exposure and improve patient response to chemoradiation. To test these hypotheses, we first examined the effects of opaganib on the toxicity and antitumor activity of radiation in mice exposed to total body irradiation (TBI) or IR with partial bone marrow shielding. Oral treatment with opaganib 2 h before TBI shifted the LD75 from 9.5 Gy to 11.5 Gy, and provided substantial protection against gastrointestinal damage associated with suppression of radiation-induced elevations of S1P and TNFα in the small intestines. In the partially shielded model, opaganib provided dose-dependent survival advantages when administered 4 h before or 24 h after radiation exposure, and was particularly effective when given both prior to and following radiation. Relevant to cancer radiotherapy, opaganib decreased the sensitivity of IEC6 (non-transformed mouse intestinal epithelial) cells to radiation, while sensitizing PAN02 cells to in vitro radiation. Next, the in vivo effects of opaganib in combination with radiation were examined in a syngeneic tumor model consisting of C57BL/6 mice bearing xenografts of PAN02 pancreatic cancer cells and a cross-species xenograft model consisting of nude mice bearing xenografts of human FaDu cells. Mice were treated with opaganib and/or IR (plus cisplatin in the case of FaDu tumors). In both tumor models, the optimal suppression of tumor growth was attained by the combination of opaganib with IR (± cisplatin). Overall, opaganib substantially protects normal tissue from radiation damage that may occur through unintended exposure or cancer radiotherapy.
Keywords: ABC294640; opaganib; radiation; sphingolipid; sphingosine kinase; xenograft.
Conflict of interest statement
Lynn W. Maines, Randy S. Schrecengost, Yan Zhuang, Staci N. Keller, Ryan A. Smith, Cecelia L. Green and Charles D. Smith were employees at the time of the research and own stock in Apogee Biotechnology Corporation. Apogee Biotechnology Corporation owns patent rights to opaganib, and the value of these rights may be affected by the research reported in the enclosed paper. The funders (BARDA and the Department of Defense) assisted and approved the design of the experiments shown in Figure 4, Figure 5, Figure 6 and Figure 7, but had no role in the collection, analyses or interpretation of the data, in the writing of the manuscript, or in the decision to publish the results.
Figures
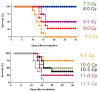
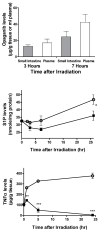
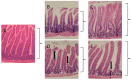
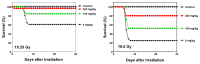
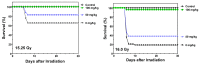
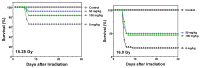
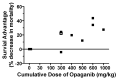
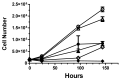
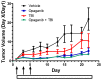
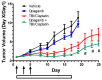
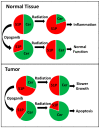
Similar articles
-
Opaganib (ABC294640) Induces Immunogenic Tumor Cell Death and Enhances Checkpoint Antibody Therapy.Int J Mol Sci. 2023 Nov 29;24(23):16901. doi: 10.3390/ijms242316901. Int J Mol Sci. 2023. PMID: 38069222 Free PMC article.
-
The Sphingolipid-Modulating Drug Opaganib Protects against Radiation-Induced Lung Inflammation and Fibrosis: Potential Uses as a Medical Countermeasure and in Cancer Radiotherapy.Int J Mol Sci. 2024 Feb 15;25(4):2322. doi: 10.3390/ijms25042322. Int J Mol Sci. 2024. PMID: 38396999 Free PMC article.
-
The Sphingosine Kinase 2 Inhibitor Opaganib Protects Against Acute Kidney Injury in Mice.Int J Nephrol Renovasc Dis. 2022 Nov 17;15:323-334. doi: 10.2147/IJNRD.S386396. eCollection 2022. Int J Nephrol Renovasc Dis. 2022. PMID: 36420520 Free PMC article.
-
Opaganib Downregulates N-Myc Expression and Suppresses In Vitro and In Vivo Growth of Neuroblastoma Cells.Cancers (Basel). 2024 May 5;16(9):1779. doi: 10.3390/cancers16091779. Cancers (Basel). 2024. PMID: 38730731 Free PMC article.
-
Recent Progress in the Development of Opaganib for the Treatment of Covid-19.Drug Des Devel Ther. 2022 Jul 12;16:2199-2211. doi: 10.2147/DDDT.S367612. eCollection 2022. Drug Des Devel Ther. 2022. PMID: 35855741 Free PMC article. Review.
Cited by
-
Opaganib (ABC294640) Induces Immunogenic Tumor Cell Death and Enhances Checkpoint Antibody Therapy.Int J Mol Sci. 2023 Nov 29;24(23):16901. doi: 10.3390/ijms242316901. Int J Mol Sci. 2023. PMID: 38069222 Free PMC article.
-
Role of Sphingosine-1-Phosphate Signaling Pathway in Pancreatic Diseases.Int J Mol Sci. 2024 Oct 25;25(21):11474. doi: 10.3390/ijms252111474. Int J Mol Sci. 2024. PMID: 39519028 Free PMC article. Review.
-
Sphingolipid-Induced Bone Regulation and Its Emerging Role in Dysfunction Due to Disease and Infection.Int J Mol Sci. 2024 Mar 5;25(5):3024. doi: 10.3390/ijms25053024. Int J Mol Sci. 2024. PMID: 38474268 Free PMC article. Review.
-
The Sphingolipid-Modulating Drug Opaganib Protects against Radiation-Induced Lung Inflammation and Fibrosis: Potential Uses as a Medical Countermeasure and in Cancer Radiotherapy.Int J Mol Sci. 2024 Feb 15;25(4):2322. doi: 10.3390/ijms25042322. Int J Mol Sci. 2024. PMID: 38396999 Free PMC article.
References
MeSH terms
Substances
Grants and funding
LinkOut - more resources
Full Text Sources
Research Materials