Advancing mRNA technologies for therapies and vaccines: An African context
- PMID: 36353641
- PMCID: PMC9637871
- DOI: 10.3389/fimmu.2022.1018961
Advancing mRNA technologies for therapies and vaccines: An African context
Abstract
Synthetic mRNA technologies represent a versatile platform that can be used to develop advanced drug products. The remarkable speed with which vaccine development programs designed and manufactured safe and effective COVID-19 vaccines has rekindled interest in mRNA technology, particularly for future pandemic preparedness. Although recent R&D has focused largely on advancing mRNA vaccines and large-scale manufacturing capabilities, the technology has been used to develop various immunotherapies, gene editing strategies, and protein replacement therapies. Within the mRNA technologies toolbox lie several platforms, design principles, and components that can be adapted to modulate immunogenicity, stability, in situ expression, and delivery. For example, incorporating modified nucleotides into conventional mRNA transcripts can reduce innate immune responses and improve in situ translation. Alternatively, self-amplifying RNA may enhance vaccine-mediated immunity by increasing antigen expression. This review will highlight recent advances in the field of synthetic mRNA therapies and vaccines, and discuss the ongoing global efforts aimed at reducing vaccine inequity by establishing mRNA manufacturing capacity within Africa and other low- and middle-income countries.
Keywords: African vaccine development; lipid nanoparticles; lyophilization; mRNA; mRNA immunogenicity; mRNA modifications; mRNA vaccines; saRNA.
Copyright © 2022 Kairuz, Samudh, Ely, Arbuthnot and Bloom.
Conflict of interest statement
AE, PA and KB are contracting partners of Afrigen Biologics and Vaccines through the mRNA Hub. The remaining authors declare that the research was conducted in the absence of any commercial or financial relationships that could be construed as a potential conflict of interest.
Figures
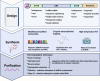
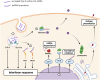
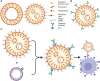
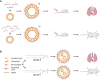
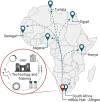
Similar articles
-
Vaccines on demand, part II: future reality.Expert Opin Drug Discov. 2023 Feb;18(2):119-127. doi: 10.1080/17460441.2022.2147501. Epub 2022 Nov 23. Expert Opin Drug Discov. 2023. PMID: 36384351 Review.
-
Synthetic modified messenger RNA for therapeutic applications.Acta Biomater. 2021 Sep 1;131:1-15. doi: 10.1016/j.actbio.2021.06.020. Epub 2021 Jun 13. Acta Biomater. 2021. PMID: 34133982 Free PMC article. Review.
-
The Rapid Development and Early Success of Covid 19 Vaccines Have Raised Hopes for Accelerating the Cancer Treatment Mechanism.Arch Razi Inst. 2021 Mar;76(1):1-6. doi: 10.22092/ari.2021.353761.1612. Epub 2021 Mar 1. Arch Razi Inst. 2021. PMID: 33818952 Free PMC article.
-
[New Hopes in Vaccine Technology: mRNA Vaccines].Mikrobiyol Bul. 2021 Apr;55(2):265-284. doi: 10.5578/mb.20219912. Mikrobiyol Bul. 2021. PMID: 33882657 Review. Turkish.
-
The Future of Epidemic and Pandemic Vaccines to Serve Global Public Health Needs.Vaccines (Basel). 2023 Mar 17;11(3):690. doi: 10.3390/vaccines11030690. Vaccines (Basel). 2023. PMID: 36992275 Free PMC article. Review.
Cited by
-
Recent Advances in Messenger Ribonucleic Acid (mRNA) Vaccines and Their Delivery Systems: A Review.Clin Pharmacol. 2023 Aug 3;15:77-98. doi: 10.2147/CPAA.S418314. eCollection 2023. Clin Pharmacol. 2023. PMID: 37554660 Free PMC article. Review.
-
Advancements in monkeypox vaccines development: a critical review of emerging technologies.Front Immunol. 2024 Oct 11;15:1456060. doi: 10.3389/fimmu.2024.1456060. eCollection 2024. Front Immunol. 2024. PMID: 39464881 Free PMC article. Review.
-
Complete substitution with modified nucleotides in self-amplifying RNA suppresses the interferon response and increases potency.Nat Biotechnol. 2024 Jul 8:10.1038/s41587-024-02306-z. doi: 10.1038/s41587-024-02306-z. Online ahead of print. Nat Biotechnol. 2024. PMID: 38977924
-
Production, Characterization, and Assessment of Permanently Cationic and Ionizable Lipid Nanoparticles for Use in the Delivery of Self-Amplifying RNA Vaccines.Pharmaceutics. 2023 Apr 7;15(4):1173. doi: 10.3390/pharmaceutics15041173. Pharmaceutics. 2023. PMID: 37111658 Free PMC article.
-
Biodrug Delivery Systems: Do mRNA Lipid Nanoparticles Come of Age?Int J Mol Sci. 2023 Jan 22;24(3):2218. doi: 10.3390/ijms24032218. Int J Mol Sci. 2023. PMID: 36768539 Free PMC article. Review.
References
Publication types
MeSH terms
Substances
LinkOut - more resources
Full Text Sources
Other Literature Sources
Medical