TSPO expression in a Zika virus murine infection model as an imaging target for acute infection-induced neuroinflammation
- PMID: 36348095
- PMCID: PMC9852192
- DOI: 10.1007/s00259-022-06019-w
TSPO expression in a Zika virus murine infection model as an imaging target for acute infection-induced neuroinflammation
Abstract
Introduction: Zika virus (ZIKV) is a neurotropic human pathogen that causes neuroinflammation, whose hallmark is elevated translocator protein (TSPO) expression in the brain. This study investigates ZIKV-associated changes in adult brain TSPO expression, evaluates the effectiveness of TSPO radioligands in detecting TSPO expression, and identifies cells that drive brain TSPO expression in a mouse infection model.
Methods: The interferon-deficient AG129 mouse infected with ZIKV was used as neuroinflammation model. TSPO expression was evaluated by tissue immunostaining. TSPO radioligands, [3H]PK11195 and [18F]FEPPA, were used for in vitro and ex vivo detection of TSPO in infected brains. [18F]FEPPA-PET was used for in vivo detection of TSPO expression. Cell subsets that contribute to TSPO expression were identified by flow cytometry.
Results: Brain TSPO expression increased with ZIKV disease severity. This increase was contributed by TSPO-positive microglia and infiltrating monocytes; and by influx of TSPO-expressing immune cells into the brain. [3H]PK11195 and [18F]FEPPA distinguish ZIKV-infected brains from normal controls in vitro and ex vivo. [18F]FEPPA brain uptake by PET imaging correlated with disease severity and neuroinflammation. However, TSPO expression by immune cells contributed to significant blood pool [18F]FEPPA activity which could confound [18F]FEPPA-PET imaging results.
Conclusions: TSPO is a biologically relevant imaging target for ZIKV neuroinflammation. Brain [18F]FEPPA uptake can be a surrogate marker for ZIKV disease and may be a potential PET imaging marker for ZIKV-induced neuroinflammation. Future TSPO-PET/SPECT studies on viral neuroinflammation and related encephalitis should assess the contribution of immune cells on TSPO expression and employ appropriate image correction methods to subtract blood pool activity.
Keywords: Neuroinflammation; PET; TSPO; Translocator protein; Zika.
© 2022. The Author(s).
Conflict of interest statement
The authors declare no competing interests.
Figures
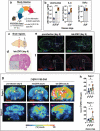
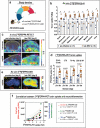
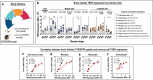
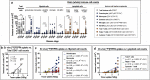
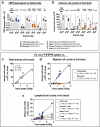
Similar articles
-
Translocator protein (TSPO) is a biomarker of Zika virus (ZIKV) infection-associated neuroinflammation.Emerg Microbes Infect. 2024 Dec;13(1):2348528. doi: 10.1080/22221751.2024.2348528. Epub 2024 May 27. Emerg Microbes Infect. 2024. PMID: 38662785 Free PMC article.
-
[18F]DPA-714 PET Imaging Reveals Global Neuroinflammation in Zika Virus-Infected Mice.Mol Imaging Biol. 2018 Apr;20(2):275-283. doi: 10.1007/s11307-017-1118-2. Mol Imaging Biol. 2018. PMID: 28900831 Free PMC article.
-
Neuroinflammation in healthy aging: a PET study using a novel Translocator Protein 18kDa (TSPO) radioligand, [(18)F]-FEPPA.Neuroimage. 2014 Jan 1;84:868-75. doi: 10.1016/j.neuroimage.2013.09.021. Epub 2013 Sep 21. Neuroimage. 2014. PMID: 24064066 Free PMC article.
-
Microglial Positron Emission Tomography Imaging In Vivo : Positron Emission Tomography Radioligands: Utility in Research and Clinical Practice.Adv Neurobiol. 2024;37:579-589. doi: 10.1007/978-3-031-55529-9_32. Adv Neurobiol. 2024. PMID: 39207714 Review.
-
TSPO imaging in animal models of brain diseases.Eur J Nucl Med Mol Imaging. 2021 Dec;49(1):77-109. doi: 10.1007/s00259-021-05379-z. Epub 2021 Jul 10. Eur J Nucl Med Mol Imaging. 2021. PMID: 34245328 Free PMC article. Review.
Cited by
-
Repurposing of Zika virus live-attenuated vaccine (ZIKV-LAV) strains as oncolytic viruses targeting human glioblastoma multiforme cells.J Transl Med. 2024 Feb 2;22(1):126. doi: 10.1186/s12967-024-04930-4. J Transl Med. 2024. PMID: 38308299 Free PMC article.
-
Translocator protein (TSPO) is a biomarker of Zika virus (ZIKV) infection-associated neuroinflammation.Emerg Microbes Infect. 2024 Dec;13(1):2348528. doi: 10.1080/22221751.2024.2348528. Epub 2024 May 27. Emerg Microbes Infect. 2024. PMID: 38662785 Free PMC article.
References
-
- Dick GW, Kitchen SF, Haddow AJ. Zika virus. I. Isolations and serological specificity. Trans R Soc Trop Med Hyg. 1952;46:509–20. 10.1016/0035-9203(52)90042-4. - PubMed
MeSH terms
Substances
Grants and funding
- H18/01/a0/018/Singapore Health and Biomedical Sciences (HBMS) Industry Alignment Fund Pre-Positioning (IAF-PP)
- AG/CIV/GC70-C/NRF/2013/3/National Research Foundation Singapore
- MOH-000524/National Medical Research Council (SG) Open Fund Individual Research Grant (OF-IRG)
- 2018/0010A/Duke-NUS Khoo Collaborative Pilot Award
LinkOut - more resources
Full Text Sources
Medical