Contrasting effects of Ksr2, an obesity gene, on trabecular bone volume and bone marrow adiposity
- PMID: 36342465
- PMCID: PMC9640193
- DOI: 10.7554/eLife.82810
Contrasting effects of Ksr2, an obesity gene, on trabecular bone volume and bone marrow adiposity
Abstract
Pathological obesity and its complications are associated with an increased propensity for bone fractures. Humans with certain genetic polymorphisms at the kinase suppressor of ras2 (KSR2) locus develop severe early-onset obesity and type 2 diabetes. Both conditions are phenocopied in mice with Ksr2 deleted, but whether this affects bone health remains unknown. Here we studied the bones of global Ksr2 null mice and found that Ksr2 negatively regulates femoral, but not vertebral, bone mass in two genetic backgrounds, while the paralogous gene, Ksr1, was dispensable for bone homeostasis. Mechanistically, KSR2 regulates bone formation by influencing adipocyte differentiation at the expense of osteoblasts in the bone marrow. Compared with Ksr2's known role as a regulator of feeding by its function in the hypothalamus, pair-feeding and osteoblast-specific conditional deletion of Ksr2 reveals that Ksr2 can regulate bone formation autonomously. Despite the gains in appendicular bone mass observed in the absence of Ksr2, bone strength, as well as fracture healing response, remains compromised in these mice. This study highlights the interrelationship between adiposity and bone health and provides mechanistic insights into how Ksr2, an adiposity and diabetic gene, regulates bone metabolism.
Keywords: adipose tissue; genetics; genomics; mouse; mouse models; osteoporosis.
Plain language summary
Our bones are living tissues which constantly reshape and renew themselves. This ability relies on stem cells present in the marrow cavity, which can mature into the various types of cells needed to produce new bone material, marrow fat, or other components. Obesity and associated conditions such as type 2 diabetes are often linked to harmful changes in the skeleton. In particular, these metabolic conditions are associated with weight-bearing bones becoming more prone to facture and healing poorly. Mice genetically modified to model obesity and diabetes could help researchers to study exactly how these conditions – and the genetic changes that underlie them – impact bone health. Gomez et al. aimed to address this question by focusing on KSR2, a gene involved in energy consumption and feeding behavior. Children who carry certain KSR2 mutations are prone to obesity and type 2 diabetes; mice lacking the gene also develop these conditions due to uncontrolled eating. Closely examining mutant mice in which Ksr2 had been deactivated in every cell revealed that the weight-bearing bones of these animals were also more likely to break, and the fractures then healed more slowly. This was the case even though these bones had higher mass and less marrow fat compared to healthy mice. Non-weight bearing bones (such as the spine) did not exhibit these changes. Further experiments revealed that, when expressed normally in the skeleton, Ksr2 skews the stem cell maturation process towards marrow fat cells instead of bone-creating cells. This suggests a new role for Ksr2, which therefore seems to independently regulate both feeding behavior and bone health. In addition, the work by Gomez et al. demonstrate that Ksr2 mutant mice could be a useful model to better understand how obesity and diabetes affect human bones, and to potentially develop new therapies.
Conflict of interest statement
GG, CR, WX, CK, SP, RL No competing interests declared, DP was employed by Lexicon Pharmaceuticals, Inc, at the time some of these studies were performed and may own common stock or have been granted stock options, SM Reviewing editor, eLife
Figures
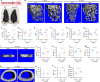
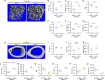
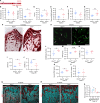
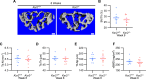
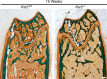

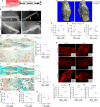
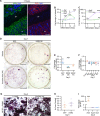
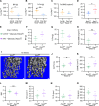
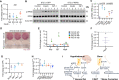
Similar articles
-
Profound obesity secondary to hyperphagia in mice lacking kinase suppressor of ras 2.Obesity (Silver Spring). 2011 May;19(5):1010-8. doi: 10.1038/oby.2010.282. Epub 2010 Dec 2. Obesity (Silver Spring). 2011. PMID: 21127480
-
CCAAT/enhancer binding protein β-deficiency enhances type 1 diabetic bone phenotype by increasing marrow adiposity and bone resorption.Am J Physiol Regul Integr Comp Physiol. 2011 May;300(5):R1250-60. doi: 10.1152/ajpregu.00764.2010. Epub 2011 Feb 23. Am J Physiol Regul Integr Comp Physiol. 2011. PMID: 21346244 Free PMC article.
-
Kinase Suppressor of Ras 2 (KSR2) expression in the brain regulates energy balance and glucose homeostasis.Mol Metab. 2016 Dec 18;6(2):194-205. doi: 10.1016/j.molmet.2016.12.004. eCollection 2017 Feb. Mol Metab. 2016. PMID: 28180061 Free PMC article.
-
The pathophysiology of osteoporosis in obesity and type 2 diabetes in aging women and men: The mechanisms and roles of increased bone marrow adiposity.Front Endocrinol (Lausanne). 2022 Sep 15;13:981487. doi: 10.3389/fendo.2022.981487. eCollection 2022. Front Endocrinol (Lausanne). 2022. PMID: 36187112 Free PMC article. Review.
-
Mechanisms of marrow adiposity and its implications for skeletal health.Metabolism. 2017 Feb;67:106-114. doi: 10.1016/j.metabol.2016.11.013. Epub 2016 Nov 27. Metabolism. 2017. PMID: 28081773 Free PMC article. Review.
Cited by
-
KSR1 Knockout Mouse Model Demonstrates MAPK Pathway's Key Role in Cisplatin- and Noise-induced Hearing Loss.J Neurosci. 2024 May 1;44(18):e2174232024. doi: 10.1523/JNEUROSCI.2174-23.2024. J Neurosci. 2024. PMID: 38548338 Free PMC article.
-
KSR1 knockout mouse model demonstrates MAPK pathway's key role in cisplatin- and noise-induced hearing loss.bioRxiv [Preprint]. 2023 Nov 13:2023.11.08.566316. doi: 10.1101/2023.11.08.566316. bioRxiv. 2023. Update in: J Neurosci. 2024 May 1;44(18):e2174232024. doi: 10.1523/JNEUROSCI.2174-23.2024 PMID: 38014104 Free PMC article. Updated. Preprint.
-
Evaluation of Potential Roles of Zinc Finger Homeobox 3 (Zfhx3) Expressed in Chondrocytes and Osteoblasts on Skeletal Growth in Mice.Calcif Tissue Int. 2024 Oct;115(4):445-454. doi: 10.1007/s00223-024-01265-6. Epub 2024 Aug 1. Calcif Tissue Int. 2024. PMID: 39085428
-
Identification of KSR2 Variants in Pediatric Patients with Severe Early-Onset Obesity from Qatar.Genes (Basel). 2024 Jul 23;15(8):966. doi: 10.3390/genes15080966. Genes (Basel). 2024. PMID: 39202327 Free PMC article.
References
-
- Aaron N, Kraakman MJ, Zhou Q, Liu Q, Costa S, Yang J, Liu L, Yu L, Wang L, He Y, Fan L, Hirakawa H, Ding L, Lo J, Wang W, Zhao B, Guo E, Sun L, Rosen CJ, Qiang L. Adipsin promotes bone marrow adiposity by priming mesenchymal stem cells. eLife. 2021;10:e69209. doi: 10.7554/eLife.69209. - DOI - PMC - PubMed
-
- Abe I, Ochi K, Takashi Y, Yamao Y, Ohishi H, Fujii H, Minezaki M, Sugimoto K, Kudo T, Abe M, Ohnishi Y, Mukoubara S, Kobayashi K. Effect of denosumab, a human monoclonal antibody of receptor activator of nuclear factor kappa-B ligand (RANKL), upon glycemic and metabolic parameters: effect of denosumab on glycemic parameters. Medicine. 2019;98:e18067. doi: 10.1097/MD.0000000000018067. - DOI - PMC - PubMed
Publication types
MeSH terms
Substances
Grants and funding
LinkOut - more resources
Full Text Sources
Molecular Biology Databases
Research Materials