Kinesin-2 motors differentially impact biogenesis of extracellular vesicle subpopulations shed from sensory cilia
- PMID: 36304122
- PMCID: PMC9593189
- DOI: 10.1016/j.isci.2022.105262
Kinesin-2 motors differentially impact biogenesis of extracellular vesicle subpopulations shed from sensory cilia
Abstract
Extracellular vesicles (EVs) are bioactive lipid-bilayer enclosed particles released from nearly all cells. One specialized site for EV shedding is the primary cilium. Here, we discover the conserved ion channel CLHM-1 as a ciliary EV cargo. Imaging of EVs released from sensory neuron cilia of Caenorhabditis elegans expressing fluorescently tagged CLHM-1 and TRP polycystin-2 channel PKD-2 shows enrichment of these cargoes in distinct EV subpopulations that are differentially shed in response to mating partner availability. PKD-2 alone is present in EVs shed from the cilium distal tip, whereas CLHM-1 EVs bud from a secondary site(s), including the ciliary base. Heterotrimeric and homodimeric kinesin-2 motors have discrete impacts on PKD-2 and CLHM-1 colocalization in both cilia and EVs. Total loss of kinesin-2 activity decreases shedding of PKD-2 but not CLHM-1 EVs. Our data demonstrate that anterograde intraflagellar transport is required for selective enrichment of protein cargoes into heterogeneous EVs with different signaling potentials.
Keywords: Molecular neuroscience; Natural sciences; Neuroscience.
© 2022 The Authors.
Conflict of interest statement
The authors declare no competing interests.
Figures
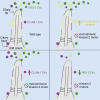
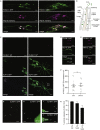
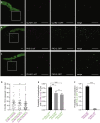
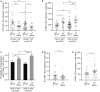
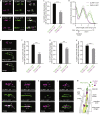
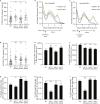
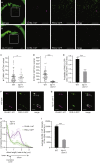
Similar articles
-
Sensory cilia act as a specialized venue for regulated extracellular vesicle biogenesis and signaling.Curr Biol. 2021 Sep 13;31(17):3943-3951.e3. doi: 10.1016/j.cub.2021.06.040. Epub 2021 Jul 15. Curr Biol. 2021. PMID: 34270950 Free PMC article.
-
Ciliary intrinsic mechanisms regulate dynamic ciliary extracellular vesicle release from sensory neurons.Curr Biol. 2024 Jun 17;34(12):2756-2763.e2. doi: 10.1016/j.cub.2024.05.015. Epub 2024 Jun 4. Curr Biol. 2024. PMID: 38838665
-
Glutamylation Regulates Transport, Specializes Function, and Sculpts the Structure of Cilia.Curr Biol. 2017 Nov 20;27(22):3430-3441.e6. doi: 10.1016/j.cub.2017.09.066. Epub 2017 Nov 9. Curr Biol. 2017. PMID: 29129530 Free PMC article.
-
Ciliary Extracellular Vesicles: Txt Msg Organelles.Cell Mol Neurobiol. 2016 Apr;36(3):449-57. doi: 10.1007/s10571-016-0345-4. Epub 2016 Mar 17. Cell Mol Neurobiol. 2016. PMID: 26983828 Free PMC article. Review.
-
Mating behavior, male sensory cilia, and polycystins in Caenorhabditis elegans.Semin Cell Dev Biol. 2014 Sep;33:25-33. doi: 10.1016/j.semcdb.2014.06.001. Epub 2014 Jun 27. Semin Cell Dev Biol. 2014. PMID: 24977333 Free PMC article. Review.
Cited by
-
Isolation, profiling, and tracking of extracellular vesicle cargo in Caenorhabditis elegans.Curr Biol. 2022 May 9;32(9):1924-1936.e6. doi: 10.1016/j.cub.2022.03.005. Epub 2022 Mar 24. Curr Biol. 2022. PMID: 35334227 Free PMC article.
-
Extracellular vesicles.Genetics. 2024 Aug 7;227(4):iyae088. doi: 10.1093/genetics/iyae088. Genetics. 2024. PMID: 38884207 Free PMC article. Review.
-
Minimal information for studies of extracellular vesicles (MISEV2023): From basic to advanced approaches.J Extracell Vesicles. 2024 Feb;13(2):e12404. doi: 10.1002/jev2.12404. J Extracell Vesicles. 2024. PMID: 38326288 Free PMC article.
-
Transport and barrier mechanisms that regulate ciliary compartmentalization and ciliopathies.Nat Rev Nephrol. 2024 Feb;20(2):83-100. doi: 10.1038/s41581-023-00773-2. Epub 2023 Oct 23. Nat Rev Nephrol. 2024. PMID: 37872350 Review.
References
-
- Blacque O.E., Reardon M.J., Li C., McCarthy J., Mahjoub M.R., Ansley S.J., Badano J.L., Mah A.K., Beales P.L., Davidson W.S., et al. Loss of C. elegans BBS-7 and BBS-8 protein function results in cilia defects and compromised intraflagellar transport. Genes Dev. 2004;18:1630–1642. doi: 10.1101/gad.1194004. - DOI - PMC - PubMed
Grants and funding
LinkOut - more resources
Full Text Sources
Research Materials
Miscellaneous