Multiplexed bioluminescence imaging with a substrate unmixing platform
- PMID: 36283402
- PMCID: PMC9675729
- DOI: 10.1016/j.chembiol.2022.10.004
Multiplexed bioluminescence imaging with a substrate unmixing platform
Abstract
Bioluminescent tools can illuminate cellular features in whole organisms. Multi-component tracking remains challenging, though, owing to a lack of well-resolved probes and long imaging times. To address the need for more rapid, quantitative, and multiplexed bioluminescent readouts, we developed an analysis pipeline featuring sequential substrate administration and serial image acquisition. Light output from each luciferin is layered on top of the previous image, with minimal delay between substrate delivery. A MATLAB algorithm was written to analyze bioluminescent images generated from the rapid imaging protocol and deconvolute (i.e., unmix) signals from luciferase-luciferin pairs. Mixtures comprising three to five luciferase reporters were readily distinguished in under 50 min; this same experiment would require days using conventional workflows. We further showed that the algorithm can be used to accurately quantify luciferase levels in heterogeneous mixtures. Based on its speed and versatility, the multiplexed imaging platform will expand the scope of bioluminescence technology.
Keywords: bioluminescence; imaging; luciferase; luciferin; multiplexing; unmixing.
Copyright © 2022 Elsevier Ltd. All rights reserved.
Conflict of interest statement
Declaration of interests J.A.P. is a member of the Editorial Advisory Board for Cell Chemical Biology.
Figures
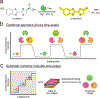
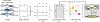
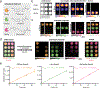
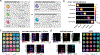
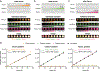
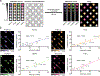
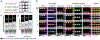
Similar articles
-
Building Biological Flashlights: Orthogonal Luciferases and Luciferins for in Vivo Imaging.Acc Chem Res. 2019 Nov 19;52(11):3039-3050. doi: 10.1021/acs.accounts.9b00391. Epub 2019 Oct 8. Acc Chem Res. 2019. PMID: 31593431 Free PMC article. Review.
-
Rapid Multicomponent Bioluminescence Imaging via Substrate Unmixing.ACS Chem Biol. 2021 Apr 16;16(4):682-690. doi: 10.1021/acschembio.0c00959. Epub 2021 Mar 17. ACS Chem Biol. 2021. PMID: 33729750 Free PMC article.
-
Multicomponent Bioluminescence Imaging with a π-Extended Luciferin.J Am Chem Soc. 2020 Aug 19;142(33):14080-14089. doi: 10.1021/jacs.0c01064. Epub 2020 Aug 4. J Am Chem Soc. 2020. PMID: 32787261 Free PMC article.
-
Multiplexed bioluminescence microscopy via phasor analysis.Nat Methods. 2022 Jul;19(7):893-898. doi: 10.1038/s41592-022-01529-9. Epub 2022 Jun 23. Nat Methods. 2022. PMID: 35739310
-
Seeing (and Using) the Light: Recent Developments in Bioluminescence Technology.Cell Chem Biol. 2020 Aug 20;27(8):904-920. doi: 10.1016/j.chembiol.2020.07.022. Epub 2020 Aug 13. Cell Chem Biol. 2020. PMID: 32795417 Free PMC article. Review.
Cited by
-
Optimized in vivo multispectral bioluminescent imaging of tumor biology using engineered BRET reporters.iScience. 2024 Aug 8;27(9):110655. doi: 10.1016/j.isci.2024.110655. eCollection 2024 Sep 20. iScience. 2024. PMID: 39252965 Free PMC article.
-
Bright Red Bioluminescence from Semisynthetic NanoLuc (sNLuc).ACS Chem Biol. 2024 May 17;19(5):1035-1039. doi: 10.1021/acschembio.4c00033. Epub 2024 May 8. ACS Chem Biol. 2024. PMID: 38717306 Free PMC article.
-
Destabilized reporters for background-subtracted, chemically-gated, and multiplexed deep-tissue imaging.Chem Sci. 2024 Jun 4;15(28):11108-11121. doi: 10.1039/d4sc00377b. eCollection 2024 Jul 17. Chem Sci. 2024. PMID: 39027298 Free PMC article.
-
Red-Shifted Coumarin Luciferins for Improved Bioluminescence Imaging.J Am Chem Soc. 2023 Feb 15;145(6):3335-3345. doi: 10.1021/jacs.2c07220. Epub 2023 Feb 6. J Am Chem Soc. 2023. PMID: 36745536 Free PMC article.
-
Caged luciferins enable rapid multicomponent bioluminescence imaging.Photochem Photobiol. 2024 Jan-Feb;100(1):67-74. doi: 10.1111/php.13814. Epub 2023 May 31. Photochem Photobiol. 2024. PMID: 37259257 Free PMC article.
References
-
- Chaincy K, Olivier C, Tamara LT, Heng X, and Bradley WR (2007). Three-dimensional reconstruction of in vivo bioluminescent sources based on multispectral imaging. J. Biomed. Opt. 12, 1–12. - PubMed