Construction and Ion Transport-Related Applications of the Hydrogel-Based Membrane with 3D Nanochannels
- PMID: 36235985
- PMCID: PMC9571189
- DOI: 10.3390/polym14194037
Construction and Ion Transport-Related Applications of the Hydrogel-Based Membrane with 3D Nanochannels
Abstract
Hydrogel is a type of crosslinked three-dimensional polymer network structure gel. It can swell and hold a large amount of water but does not dissolve. It is an excellent membrane material for ion transportation. As transport channels, the chemical structure of hydrogel can be regulated by molecular design, and its three-dimensional structure can be controlled according to the degree of crosslinking. In this review, our prime focus has been on ion transport-related applications based on hydrogel materials. We have briefly elaborated the origin and source of hydrogel materials and summarized the crosslinking mechanisms involved in matrix network construction and the different spatial network structures. Hydrogel structure and the remarkable performance features such as microporosity, ion carrying capability, water holding capacity, and responsiveness to stimuli such as pH, light, temperature, electricity, and magnetic field are discussed. Moreover, emphasis has been made on the application of hydrogels in water purification, energy storage, sensing, and salinity gradient energy conversion. Finally, the prospects and challenges related to hydrogel fabrication and applications are summarized.
Keywords: 3D structure; hydrogels; ion channels.
Conflict of interest statement
The authors declare no conflict of interest.
Figures
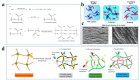
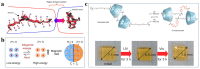
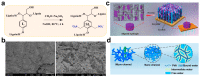
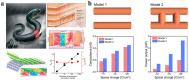
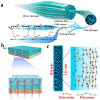
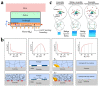
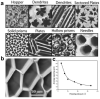
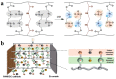
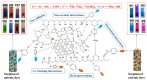
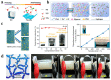
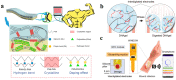
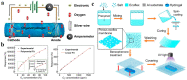
Similar articles
-
Enhanced osmotic energy conversion through bacterial cellulose based double-network hydrogel with 3D interconnected nanochannels.Carbohydr Polym. 2023 Apr 1;305:120556. doi: 10.1016/j.carbpol.2023.120556. Epub 2023 Jan 7. Carbohydr Polym. 2023. PMID: 36737202
-
Exploiting the role of nanoparticles for use in hydrogel-based bioprinting applications: concept, design, and recent advances.Biomater Sci. 2021 Sep 28;9(19):6337-6354. doi: 10.1039/d1bm00605c. Biomater Sci. 2021. PMID: 34397056 Review.
-
Programmable Morphing Hydrogels for Soft Actuators and Robots: From Structure Designs to Active Functions.Acc Chem Res. 2022 Jun 7;55(11):1533-1545. doi: 10.1021/acs.accounts.2c00046. Epub 2022 Apr 12. Acc Chem Res. 2022. PMID: 35413187
-
Rational ion transport management mediated through membrane structures.Exploration (Beijing). 2021 Oct 30;1(2):20210101. doi: 10.1002/EXP.20210101. eCollection 2021 Oct. Exploration (Beijing). 2021. PMID: 37323215 Free PMC article. Review.
-
Light-Controlled Ionic/Molecular Transport through Solid-State Nanopores and Nanochannels.Chem Asian J. 2022 May 16;17(10):e202200158. doi: 10.1002/asia.202200158. Epub 2022 Apr 20. Chem Asian J. 2022. PMID: 35324076 Review.
Cited by
-
State-of-the-Art Advances and Current Applications of Gel-Based Membranes.Gels. 2024 Jan 1;10(1):39. doi: 10.3390/gels10010039. Gels. 2024. PMID: 38247761 Free PMC article. Review.
-
3D cell culture model: From ground experiment to microgravity study.Front Bioeng Biotechnol. 2023 Mar 24;11:1136583. doi: 10.3389/fbioe.2023.1136583. eCollection 2023. Front Bioeng Biotechnol. 2023. PMID: 37034251 Free PMC article. Review.
-
Dual-Temperature/pH-Sensitive Hydrogels with Excellent Strength and Toughness Crosslinked Using Three Crosslinking Methods.Gels. 2024 Jul 19;10(7):480. doi: 10.3390/gels10070480. Gels. 2024. PMID: 39057503 Free PMC article.
References
-
- Yang C., Suo Z. Hydrogel ionotronics. Nat. Rev. Mater. 2018;3:125–142. doi: 10.1038/s41578-018-0018-7. - DOI
-
- Leng K., Li G., Guo J., Zhang X., Wang A., Liu X., Luo J. A Safe Polyzwitterionic Hydrogel Electrolyte for Long-Life Quasi-Solid State Zinc Metal Batteries. Adv. Funct. Mater. 2020;30:2001317. doi: 10.1002/adfm.202001317. - DOI
-
- Wichterle O., Lim D. Hydrophilic Gels for Biological Use. Nature. 1960;185:117–118. doi: 10.1038/185117a0. - DOI
Publication types
Grants and funding
LinkOut - more resources
Full Text Sources
Research Materials