Drosophila as a Model Organism to Study Basic Mechanisms of Longevity
- PMID: 36232546
- PMCID: PMC9569508
- DOI: 10.3390/ijms231911244
Drosophila as a Model Organism to Study Basic Mechanisms of Longevity
Abstract
The spatio-temporal regulation of gene expression determines the fate and function of various cells and tissues and, as a consequence, the correct development and functioning of complex organisms. Certain mechanisms of gene activity regulation provide adequate cell responses to changes in environmental factors. Aside from gene expression disorders that lead to various pathologies, alterations of expression of particular genes were shown to significantly decrease or increase the lifespan in a wide range of organisms from yeast to human. Drosophila fruit fly is an ideal model system to explore mechanisms of longevity and aging due to low cost, easy handling and maintenance, large number of progeny per adult, short life cycle and lifespan, relatively low number of paralogous genes, high evolutionary conservation of epigenetic mechanisms and signalling pathways, and availability of a wide range of tools to modulate gene expression in vivo. Here, we focus on the organization of the evolutionarily conserved signaling pathways whose components significantly influence the aging process and on the interconnections of these pathways with gene expression regulation.
Keywords: Drosophila; IIS; JNK; NF-κB; TOR; aging; gene expression regulation; longevity; signaling pathway.
Conflict of interest statement
The authors declare no conflict of interest.
Figures
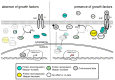
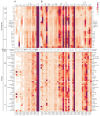
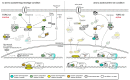
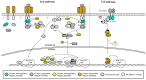
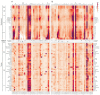
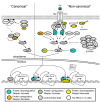
Similar articles
-
Slowed aging during reproductive dormancy is reflected in genome-wide transcriptome changes in Drosophila melanogaster.BMC Genomics. 2016 Jan 13;17:50. doi: 10.1186/s12864-016-2383-1. BMC Genomics. 2016. PMID: 26758761 Free PMC article.
-
Transcriptional feedback in the insulin signalling pathway modulates ageing in both Caenorhabditis elegans and Drosophila melanogaster.Mol Biosyst. 2013 Jul;9(7):1756-64. doi: 10.1039/c3mb25485b. Epub 2013 Apr 26. Mol Biosyst. 2013. PMID: 23624434 Free PMC article.
-
Drosophila germ-line modulation of insulin signaling and lifespan.Proc Natl Acad Sci U S A. 2008 Apr 29;105(17):6368-73. doi: 10.1073/pnas.0709128105. Epub 2008 Apr 23. Proc Natl Acad Sci U S A. 2008. PMID: 18434551 Free PMC article.
-
Role of insulin-like signalling in Drosophila lifespan.Trends Biochem Sci. 2007 Apr;32(4):180-8. doi: 10.1016/j.tibs.2007.02.007. Epub 2007 Apr 6. Trends Biochem Sci. 2007. PMID: 17412594 Review.
-
Endocrine Regulation of Aging in the Fruit Fly Drosophila melanogaster.Zoolog Sci. 2024 Feb;41(1):4-13. doi: 10.2108/zs230056. Zoolog Sci. 2024. PMID: 38587512 Review.
Cited by
-
Insect-pathogen crosstalk and the cellular-molecular mechanisms of insect immunity: uncovering the underlying signaling pathways and immune regulatory function of non-coding RNAs.Front Immunol. 2023 Aug 24;14:1169152. doi: 10.3389/fimmu.2023.1169152. eCollection 2023. Front Immunol. 2023. PMID: 37691928 Free PMC article. Review.
-
An Introductory Guide to Using Bloomington Drosophila Stock Center and FlyBase for Aging Research.Cells. 2024 Jul 14;13(14):1192. doi: 10.3390/cells13141192. Cells. 2024. PMID: 39056774 Free PMC article. Review.
-
Nutritional Programming of the Lifespan of Male Drosophila by Activating FOXO on Larval Low-Nutrient Diet.Nutrients. 2023 Apr 11;15(8):1840. doi: 10.3390/nu15081840. Nutrients. 2023. PMID: 37111059 Free PMC article.
-
Genetic, Environmental, and Stochastic Components of Lifespan Variability: The Drosophila Paradigm.Int J Mol Sci. 2024 Apr 19;25(8):4482. doi: 10.3390/ijms25084482. Int J Mol Sci. 2024. PMID: 38674068 Free PMC article. Review.
-
Angelica keiskei Impacts the Lifespan and Healthspan of Drosophila melanogaster in a Sex and Strain-Dependent Manner.Pharmaceuticals (Basel). 2023 May 12;16(5):738. doi: 10.3390/ph16050738. Pharmaceuticals (Basel). 2023. PMID: 37242522 Free PMC article.
References
Publication types
MeSH terms
Substances
Grants and funding
LinkOut - more resources
Full Text Sources
Molecular Biology Databases
Research Materials