Brain Endothelial Cells in Contrary to the Aortic Do Not Transport but Degrade Low-Density Lipoproteins via Both LDLR and ALK1
- PMID: 36231005
- PMCID: PMC9564369
- DOI: 10.3390/cells11193044
Brain Endothelial Cells in Contrary to the Aortic Do Not Transport but Degrade Low-Density Lipoproteins via Both LDLR and ALK1
Abstract
The transport of low-density lipoprotein (LDL) through the endothelium is a key step in the development of atherosclerosis, but it is notorious that phenotypic differences exist between endothelial cells originating from different vascular beds. Endothelial cells forming the blood-brain barrier restrict paracellular and transcellular passage of plasma proteins. Here, we systematically compared brain versus aortic endothelial cells towards their interaction with LDL and the role of proteins known to regulate the uptake of LDL by endothelial cells. Both brain endothelial cells and aortic endothelial cells bind and internalize LDL. However, whereas aortic endothelial cells degrade very small amounts of LDL and transcytose the majority, brain endothelial cells degrade but do not transport LDL. Using RNA interference (siRNA), we found that the LDLR-clathrin pathway leads to LDL degradation in either endothelial cell type. Both loss- and gain-of-function experiments showed that ALK1, which promotes transcellular LDL transport in aortic endothelial cells, also limits LDL degradation in brain endothelial cells. SR-BI and caveolin-1, which promote LDL uptake and transport into aortic endothelial cells, limit neither binding nor association of LDL to brain endothelial cells. Together, these results indicate distinct LDL trafficking by brain microvascular endothelial cells and aortic endothelial cells.
Keywords: Alzheimer’s disease; BBB; LDL; atherosclerosis; blood–brain barrier; endothelial cells; low-density lipoprotein.
Conflict of interest statement
The authors declare no conflict of interest in relation to the present work.
Figures
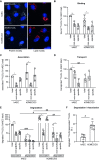
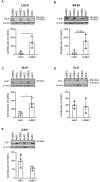
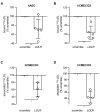
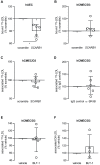
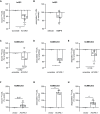
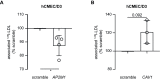
Similar articles
-
Salidroside-Mediated Autophagic Targeting of Active Src and Caveolin-1 Suppresses Low-Density Lipoprotein Transcytosis across Endothelial Cells.Oxid Med Cell Longev. 2020 Jun 23;2020:9595036. doi: 10.1155/2020/9595036. eCollection 2020. Oxid Med Cell Longev. 2020. PMID: 32685103 Free PMC article.
-
VEGF-A Regulates Cellular Localization of SR-BI as Well as Transendothelial Transport of HDL but Not LDL.Arterioscler Thromb Vasc Biol. 2017 May;37(5):794-803. doi: 10.1161/ATVBAHA.117.309284. Epub 2017 Mar 30. Arterioscler Thromb Vasc Biol. 2017. PMID: 28360088
-
Genome-wide RNAi screen reveals ALK1 mediates LDL uptake and transcytosis in endothelial cells.Nat Commun. 2016 Nov 21;7:13516. doi: 10.1038/ncomms13516. Nat Commun. 2016. PMID: 27869117 Free PMC article.
-
Transport of LDLs into the arterial wall: impact in atherosclerosis.Curr Opin Lipidol. 2020 Oct;31(5):279-285. doi: 10.1097/MOL.0000000000000701. Curr Opin Lipidol. 2020. PMID: 32773465 Free PMC article. Review.
-
LDL Transcytosis by the Arterial Endothelium-Atherosclerosis by a Thousand Cuts?Curr Atheroscler Rep. 2023 Aug;25(8):457-465. doi: 10.1007/s11883-023-01118-x. Epub 2023 Jun 26. Curr Atheroscler Rep. 2023. PMID: 37358804 Review.
Cited by
-
Caveolin-1 and Atherosclerosis: Regulation of LDLs Fate in Endothelial Cells.Int J Mol Sci. 2023 May 17;24(10):8869. doi: 10.3390/ijms24108869. Int J Mol Sci. 2023. PMID: 37240214 Free PMC article. Review.
-
Sphingosine-1-phosphate receptor 3 regulates the transendothelial transport of high-density lipoproteins and low-density lipoproteins in opposite ways.Cardiovasc Res. 2024 Apr 30;120(5):476-489. doi: 10.1093/cvr/cvad183. Cardiovasc Res. 2024. PMID: 38109696 Free PMC article.
References
-
- Kraehling J.R., Chidlow J.H., Rajagopal C., Sugiyama M.G., Fowler J.W., Lee M.Y., Zhang X., Ramírez C.M., Park E.J., Tao B., et al. Genome-Wide RNAi Screen Reveals ALK1 Mediates LDL Uptake and Transcytosis in Endothelial Cells. Nat. Commun. 2016;7:13516. doi: 10.1038/ncomms13516. - DOI - PMC - PubMed
Publication types
MeSH terms
Substances
Grants and funding
LinkOut - more resources
Full Text Sources
Research Materials