The photoprotection mechanism in the black-brown pigment eumelanin
- PMID: 36227945
- PMCID: PMC9618045
- DOI: 10.1073/pnas.2212343119
The photoprotection mechanism in the black-brown pigment eumelanin
Abstract
The natural black-brown pigment eumelanin protects humans from high-energy UV photons by absorbing and rapidly dissipating their energy before proteins and DNA are damaged. The extremely weak fluorescence of eumelanin points toward nonradiative relaxation on the timescale of picoseconds or shorter. However, the extreme chemical and physical complexity of eumelanin masks its photoprotection mechanism. We sought to determine the electronic and structural relaxation pathways in eumelanin using three complementary ultrafast optical spectroscopy methods: fluorescence, transient absorption, and stimulated Raman spectroscopies. We show that photoexcitation of chromophores across the UV-visible spectrum rapidly generates a distribution of visible excitation energies via ultrafast internal conversion among neighboring coupled chromophores, and then all these excitations relax on a timescale of ∼4 ps without transferring their energy to other chromophores. Moreover, these picosecond dynamics are shared by the monomeric building block, 5,6-dihydroxyindole-2-carboxylic acid. Through a series of solvent and pH-dependent measurements complemented by quantum chemical modeling, we show that these ultrafast dynamics are consistent with the partial excited-state proton transfer from the catechol hydroxy groups to the solvent. The use of this multispectroscopic approach allows the minimal functional unit in eumelanin and the role of exciton coupling and excited-state proton transfer to be determined, and ultimately reveals the mechanism of photoprotection in eumelanin. This knowledge has potential for use in the design of new soft optical components and organic sunscreens.
Keywords: eumelanin; excited-state proton transfer; excitons; photochemistry; ultrafast spectroscopy.
Conflict of interest statement
The authors declare no competing interest.
Figures
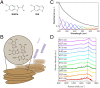
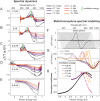
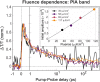
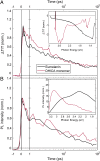
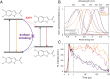
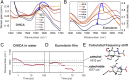
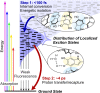
Similar articles
-
Probing the heterogeneous structure of eumelanin using ultrafast vibrational fingerprinting.Nat Commun. 2020 Sep 11;11(1):4569. doi: 10.1038/s41467-020-18393-w. Nat Commun. 2020. PMID: 32917892 Free PMC article.
-
Ultrafast spectral hole burning reveals the distinct chromophores in eumelanin and their common photoresponse.Chem Sci. 2019 Dec 18;11(5):1248-1259. doi: 10.1039/c9sc04527a. Chem Sci. 2019. PMID: 34123249 Free PMC article.
-
Role of solvent, pH, and molecular size in excited-state deactivation of key eumelanin building blocks: implications for melanin pigment photostability.J Am Chem Soc. 2008 Dec 17;130(50):17038-43. doi: 10.1021/ja806345q. J Am Chem Soc. 2008. PMID: 19007162
-
Fundamentals of the Intrinsic DNA Fluorescence.Acc Chem Res. 2021 Mar 2;54(5):1226-1235. doi: 10.1021/acs.accounts.0c00603. Epub 2021 Feb 15. Acc Chem Res. 2021. PMID: 33587613 Review.
-
Ultrafast chemistry: using time-resolved vibrational spectroscopy for interrogation of structural dynamics.Annu Rev Phys Chem. 2005;56:337-67. doi: 10.1146/annurev.physchem.56.092503.141314. Annu Rev Phys Chem. 2005. PMID: 15796704 Review.
Cited by
-
Funneling energy through disorder.Proc Natl Acad Sci U S A. 2022 Dec 6;119(49):e2216527119. doi: 10.1073/pnas.2216527119. Epub 2022 Nov 28. Proc Natl Acad Sci U S A. 2022. PMID: 36442098 Free PMC article. No abstract available.
-
Electron diffraction and solid-state NMR reveal the structure and exciton coupling in a eumelanin precursor.Chem Sci. 2024 Sep 16;15(39):16015-24. doi: 10.1039/d4sc05453a. Online ahead of print. Chem Sci. 2024. PMID: 39345764 Free PMC article.
-
Ultraviolet Superradiance from Mega-Networks of Tryptophan in Biological Architectures.J Phys Chem B. 2024 May 2;128(17):4035-4046. doi: 10.1021/acs.jpcb.3c07936. Epub 2024 Apr 19. J Phys Chem B. 2024. PMID: 38641327 Free PMC article.
-
RPE melanin and its influence on the progression of AMD.Ageing Res Rev. 2024 Aug;99:102358. doi: 10.1016/j.arr.2024.102358. Epub 2024 Jun 1. Ageing Res Rev. 2024. PMID: 38830546 Review.
-
Multiple Enol-Keto Isomerization and Excited-State Unidirectional Intramolecular Proton Transfer Generate Intense, Narrowband Red OLEDs.J Am Chem Soc. 2024 Sep 4;146(35):24526-24536. doi: 10.1021/jacs.4c07364. Epub 2024 Aug 23. J Am Chem Soc. 2024. PMID: 39177295 Free PMC article.
References
-
- d’Ischia M., et al. , Melanins and melanogenesis: From pigment cells to human health and technological applications. Pigment Cell Melanoma Res. 28, 520–544 (2015). - PubMed
-
- Huijser A., Pezzella A., Sundström V., Functionality of epidermal melanin pigments: Current knowledge on UV-dissipative mechanisms and research perspectives. Phys. Chem. Chem. Phys. 13, 9119–9127 (2011). - PubMed
-
- Meredith P., Sarna T., The physical and chemical properties of eumelanin. Pigment Cell Res. 19, 572–594 (2006). - PubMed
-
- Miannay F.-A., Bányász A., Gustavsson T., Markovitsi D., Ultrafast excited-state deactivation and energy transfer in guanine-cytosine DNA double helices. J. Am. Chem. Soc. 129, 14574–14575 (2007). - PubMed
Publication types
MeSH terms
Substances
LinkOut - more resources
Full Text Sources
Medical